A realistic bio-inspired robotic finger
October 9, 2015
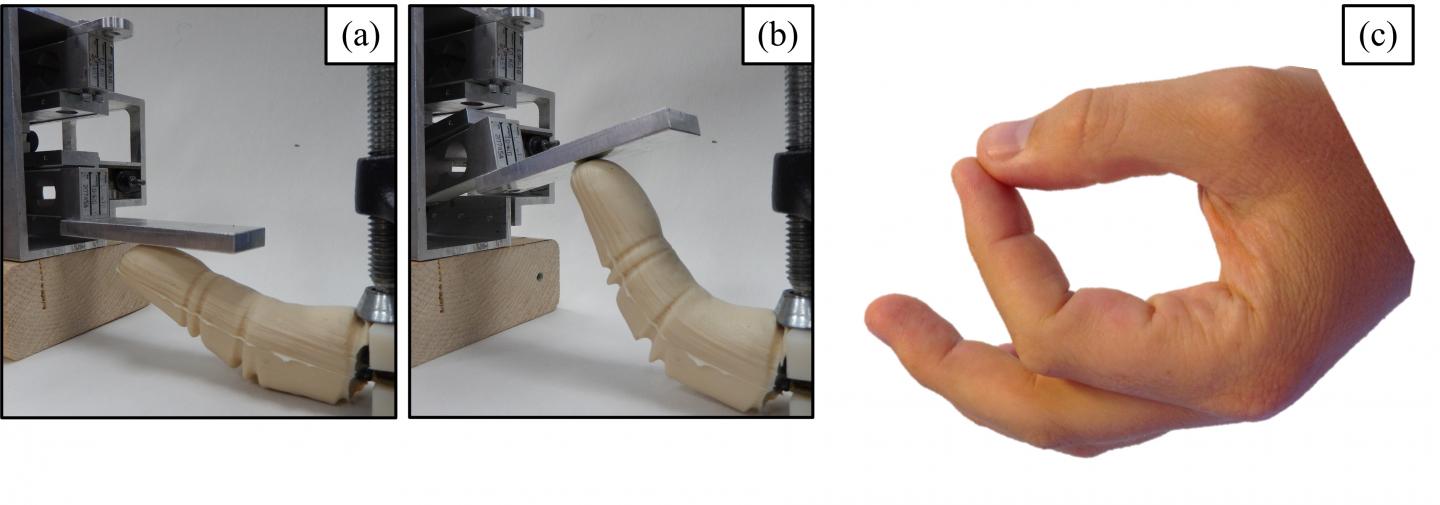
Heating and cooling a 3D-printed shape memory alloy to operate a robotic finger (credit: Florida Atlantic University/Bioinspiration & Biomimetics)
A realistic 3D-printed robotic finger using a shape memory alloy (SMA) and a unique thermal training technique has been developed by Florida Atlantic University assistant professor Erik Engeberg, Ph.D.
“We have been able to thermomechanically train our robotic finger to mimic the motions of a human finger, like flexion and extension,” said Engeberg. “Because of its light weight, dexterity and strength, our robotic design offers tremendous advantages over traditional mechanisms, and could ultimately be adapted for use as a prosthetic device, such as on a prosthetic hand.”
Most robotic parts used today are rigid, have a limited range of motion and don’t look lifelike.
In the study, described in an open-access article in the journal Bioinspiration & Biomimetics, Engeberg and his team used a resistive heating process called “Joule” heating that involves the passage of electric currents through a conductor that releases heat.
How to create a robotic finger
- The researchers first downloaded a 3-D computer-aided design (CAD) model of a human finger from the Autodesk 123D website (under creative commons license).
- With a 3-D printer, they created the inner and outer molds that housed a flexor and extensor actuator and a position sensor. The extensor actuator takes a straight shape when it’s heated and the flexor actuator takes a curved shape when heated.
- They used SMA plates and a multi-stage casting process to assemble the finger.
- Electric currents flow through each SMA actuator from an electric power source at the base of the finger as a heating and cooling process to operate the robotic finger.
Results from the study showed a rapid flexing and extending motion of the finger and ability to recover its trained shape accurately and completely, confirming the biomechanical basis of its trained shape.
Initial use in underwater robotics
“Because SMAs require a heating process and cooling process, there are challenges with this technology, such as the lengthy amount of time it takes for them to cool and return to their natural shape, even with forced air convection,” said Engeberg. So they used the technology for underwater robotics, which would provide a rapid-cooling environment.
Engeberg used thermal insulators at the fingertip, which were kept open to facilitate water flow inside the finger. As the finger flexed and extended, water flowed through the inner cavity within each insulator to cool the actuators.
“Because our robotic finger consistently recovered its thermomechanically trained shape better than other similar technologies, our underwater experiments clearly demonstrated that the water cooling component greatly increased the operational speed of the finger,” said Engeberg.
Undersea applications using Engeberg’s new technology could help to address some of the difficulties and challenges humans encounter while working in ocean depths.
FAU – BioRobotics Lab | Bottle Pick and Drop Demo UR10 and Shadow Hand
FAU – BioRobotics Lab | Simultaneous Grasp Synergies Controlled by EMG
FAU – BioRobotics Lab | Shadow Hand and UR10 – Grab Bottle, Pour Liquid
Abstract of Anthropomorphic finger antagonistically actuated by SMA plates
Most robotic applications that contain shape memory alloy (SMA) actuators use the SMA in a linear or spring shape. In contrast, a novel robotic finger was designed in this paper using SMA plates that were thermomechanically trained to take the shape of a flexed human finger when Joule heated. This flexor actuator was placed in parallel with an extensor actuator that was designed to straighten when Joule heated. Thus, alternately heating and cooling the flexor and extensor actuators caused the finger to flex and extend. Three different NiTi based SMA plates were evaluated for their ability to apply forces to a rigid and compliant object. The best of these three SMAs was able to apply a maximum fingertip force of 9.01N on average. A 3D CAD model of a human finger was used to create a solid model for the mold of the finger covering skin. Using a 3D printer, inner and outer molds were fabricated to house the actuators and a position sensor, which were assembled using a multi-stage casting process. Next, a nonlinear antagonistic controller was developed using an outer position control loop with two inner MOSFET current control loops. Sine and square wave tracking experiments demonstrated minimal errors within the operational bounds of the finger. The ability of the finger to recover from unexpected disturbances was also shown along with the frequency response up to 7 rad s−1. The closed loop bandwidth of the system was 6.4 rad s−1 when operated intermittently and 1.8 rad s−1 when operated continuously.