A step towards ‘programmable materials’
March 13, 2014
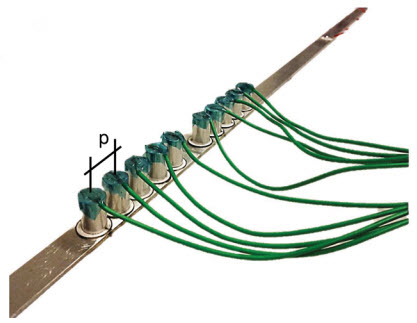
A one-dimensional working model of a programmable vibration-damping material. Each stub has a piezoelectric disc (which converts mechanical to electrical energy) connected to an external inductive circuit to damp (reduce) a specific resonance frequency. The combined stubs then create a specific frequency pass band (range). (Credit: A. Bergamini et al./Advanced Materials)
Researchers from Empa and ETH Zurich have developed a prototype of a selective vibration-damping material that they claim “could change the world of mechanics forever” as a step toward “programmable materials.”
Described in the journal Advanced Materials, this “material of the future” can damp mechanical vibrations completely or selectively suppress specific vibration frequencies or ranges of frequencies.
The one-dimensional working model consists of a simple aluminum sheet-metal strip, measuring one meter by one centimeter and one millimeter thick and designed to vibrate at different frequencies. To control the wave propagation through the plate, ten small aluminum cylinders (7 mm thick, 1 cm high) are attached to the metal.
Between the sheet and the cylinders sit piezoelectric discs, which can be stimulated electronically to instantly change their thickness.
That allows for controlling exactly how waves are allowed to propagate in the sheet-metal strip. The aluminum strip thus turns into an “adaptive phononic crystal” — a material with controllable vibration properties.
Instant adaptation
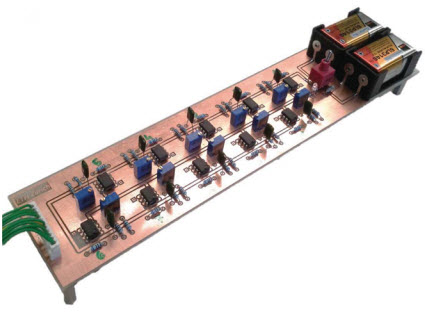
Full view of programmable vibration-damping working model, showing inductors and stubs (credit: A. Berganini et al./Advanced Materials)
The piezo controls can be set in such a way that phononic waves are able to propagate through the sheet-metal strip “perfectly normally” (as though no aluminum cylinders were attached to it); or another configuration enables a specific frequency spectrum of the waves to be absorbed.
And this muffling is variable — the piezo elements can alter their elastic properties electronically in fractions of a second — from low to high stiffness.
“Imagine you produce a sheet of metal, imprinted with an electronic circuit and small piezo elements at regular intervals,” said project supervisor Andrea Bergamini. “This sheet metal could be programmed electronically to block a certain vibration frequency. The interesting thing is that even if you cut off part of the sheet, the waves in the cropped section would largely spread in the same way as in the initial piece,” similar to a hologram.
This method could also be extended to work with three-dimensional components.
Such a “metamaterial” could fundamentally revolutionize mechanical engineering and plant construction, Bergamini says. Until now, the vibration properties were already determined in the selection of material and the geometry of the part. In the future, the material could react to current vibration readings and quickly adapt its vibration properties.
Not your ordinary shock absorber
“The way this is done is substantially different and more fundamental than applying some vibration damping treatment such as a damper or a vibration absorber,” Bergamini explained to KurzweilAI in an interview.
“By exploiting the same laws of physics that determine the transmission of heat in solid matter (crystals), we can modify the propagation of mechanical waves through structures. While this concept has been demonstrated in the purely mechanical domain by other groups (e.g., Zhengyou Liu et al. Science 289, 1734 (2000)), what is more interesting is to transduce [convert] power from the mechanical to the electrical domain, using piezoelectric materials.
“Once the power is in the electrical domain it is ‘easier’ to treat it in a way that allows for obtaining unusual behaviors, such as opening of a pass band [a specific range of frequencies] within a band gap (as presented in the Advanced Materials paper). We show that by using piezoelectric materials in the unit cell (so to say, the fundamental building block of such a macroscopic periodic structure), we can modify the effective connectivity pattern of the structure and virtually change its geometry by changing some electrical parameters.
“This is at the origin of the idea that over time one could develop ‘programmable’ materials, i.e., materials whose effective connectivity (not the physical connectivity that our eyes can see but the connectivity that affects certain properties of the structure) can be changed electrically, eventually in a computer controlled way.”
Practical applications
Bergamini said examples of uses of this new technology include suppressing vibrations in vehicles or aircraft in specific frequency ranges, microphones (suppressing rumbles, for example), headsets (noise reduction for selective frequency ranges), rocket launchers (avoid damage to sensitive devices in satellites during launch), or any precision device affected by mechanical vibrations, such as microscopes, and that these functions could be automatically adjusted in real time under computer control. (Imagine driving over a bumpy road and having the car’s shock mounts adjust their frequency response in real time to block the specific vibrations — perhaps the road of the future could transmit the frequencies of bumps based on the vehicle’s speed?)
“While the development process for new materials is typically of the order of ten years of more from lab to market, the R&D work related to this innovation is more of structural nature, as no new materials in the traditional sense (i.e. new alloys, polymers or ceramics) need to be developed in this case,” Bergamini added.
“The concept described in the papers can of course take advantage of new materials with higher performance that might be developed by other labs. We could expect that in about five years from now, some meaningful applications of these concepts could be available commercially, maybe initially on a small scale.”
Abstract of Advanced Materials paper
The band structure of a phononic crystal can be controlled by tuning the mechanical stiffness of the links connecting its constituting elements. The first implementation of a phononic crystal with adaptive connectivity is obtained by using piezoelectric resonators as variable stiffness elements, and its wave-propagation properties are experimentally characterized.