Bioelectricity found to control brain development in tadpole embryos
March 20, 2015
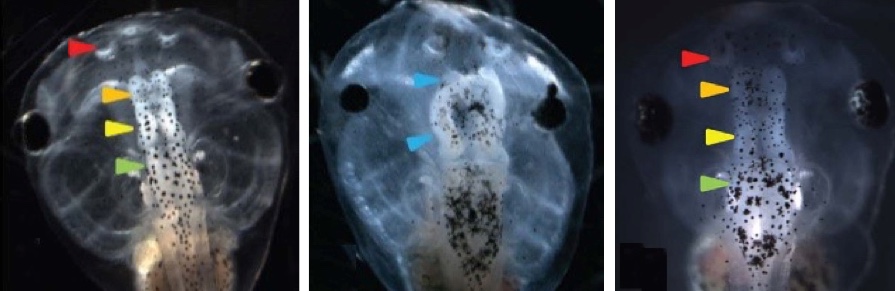
Left: normal tadpole brain. Center: injections with a suppressor of neural induction (Notch) caused a significantly elevated incidence of malformed brain in tadpole embryos, including near complete loss of forebrain/olfactory bulbs, and malformed midbrain and eyes. The embryos also exhibited loss of the normal voltage pattern. Right: Restoring hyperpolarization (normal voltage pattern) restored normal brain morphology, with well-formed forebrain/olfactory bulb, midbrain, and hindbrain. (credit: Vaibhav P. Pai et al./ The Journal of Neuroscience)
Research by Tufts University and University of Minnesota biologists shows for the first time that bioelectrical signals among cells control and guide embryonic brain development.
They also found that manipulating these signals can repair genetic defects and induce development of healthy brain tissue in locations where it would not ordinarily grow.
In their research with Xenopus laevis frog embryos (which share many evolutionary traits with humans), they found that bioelectric signaling regulates the activity of two cell reprogramming factors (proteins that can turn adult cells into stem cells).
Their results appear in the March 11, 2015 edition of The Journal of Neuroscience.
How cells use bioelectrical signals to communicate and control
“We’ve found that cells communicate, even across long distances in the embryo, using bioelectrical signals, and they use this information to know where to form a brain and how big that brain should be,” says the paper’s corresponding author Michael Levin, Ph.D., who holds the Vannevar Bush Chair in biology and directs the Center for Regenerative and Developmental Biology in the School of Arts and Sciences at Tufts University. “The signals are not just necessary for normal development; they are instructive.”
The research expands on previous work by Levin at Tufts, in which biologists were able to cause tissue to grow a new organ by simply altering the membrane voltage gradients of cells, causing tadpoles to grow eyes outside of the head area, as KurzweilAI reported in 2011.
But the concept actually dates back to 1932, when Dr. Harold S. Burr, Professor Emeritus, Anatomy at Yale University School of Medicine, found that electrical gradients across tissue can direct growth and form of organisms. He later discovered in 1937 that abnormal growth (such as cancer) was preceded by the appearance of abnormal voltage gradients in an organ.
These bioelectric signals are implemented by changes in the voltage difference across cell membranes — called the cellular resting potential — and the differential voltage (different voltage level) across anatomical regions.
Bioelectric signaling involves different cell types, including mature somatic cells and stem cells.
Overriding Genetic Defects
“This latest research also demonstrated molecular techniques for ‘hijacking’ this bioelectric communication to force the body to make new brain tissue at other locations and to fix genetic defects that cause brain malformation,” says Levin. “This means we may be able to induce growth of new brain tissue to address birth defects or brain injury, which is very exciting for regenerative medicine.”
A case in point is the Notch signaling pathway, a protein signaling system that plays a role in neural cell growth and differentiation in mammals and most other multicellular organisms. Defects in Notch signaling disrupt brain development and are also associated with disorders such as T-cell acute lymphoblastic leukemia and multiple sclerosis.
The research team found that using molecular techniques to force proper bioelectrical states in cells enabled them to override the defects induced by Notch malfunction, resulting in a much more normal brain despite a genetically defective Notch protein.
Electroceuticals
Bioelectricity and reprogramming factors work together to regulate tissue fate, says Levin. “Additional study is needed to understand more fully the mechanisms by which electric signaling interacts with genetic networks. However, here we show two such steps, involving calcium signaling and cell-cell communication via electrical synapses known as gap junctions.
“We are working on applying these techniques in biomedical contexts, especially ion channel modulating drugs — electroceuticals — to repair defects and induce brain regeneration.”
The research was supported by the National Institutes of Health, the National Science Foundation, and the G. Harold and Leila Y. Mathers Charitable Foundation.
Abstract of Endogenous Gradients of Resting Potential Instructively Pattern Embryonic Neural Tissue via Notch Signaling and Regulation of Proliferation
Biophysical forces play important roles throughout embryogenesis, but the roles of spatial differences in cellular resting potentials during large-scale brain morphogenesis remain unknown. Here, we implicate endogenous bioelectricity as an instructive factor during brain patterning in Xenopus laevis. Early frog embryos exhibit a characteristic hyperpolarization of cells lining the neural tube; disruption of this spatial gradient of the transmembrane potential (Vmem) diminishes or eliminates the expression of early brain markers, and causes anatomical mispatterning of the brain, including absent or malformed regions. This effect is mediated by voltage-gated calcium signaling and gap-junctional communication. In addition to cell-autonomous effects, we show that hyperpolarization of transmembrane potential (Vmem) in ventral cells outside the brain induces upregulation of neural cell proliferation at long range. Misexpression of the constitutively active form of Notch, a suppressor of neural induction, impairs the normal hyperpolarization pattern and neural patterning; forced hyperpolarization by misexpression of specific ion channels rescues brain defects induced by activated Notch signaling. Strikingly, hyperpolarizing posterior or ventral cells induces the production of ectopic neural tissue considerably outside the neural field. The hyperpolarization signal also synergizes with canonical reprogramming factors (POU and HB4), directing undifferentiated cells toward neural fate in vivo. These data identify a new functional role for bioelectric signaling in brain patterning, reveal interactions between Vmem and key biochemical pathways (Notch and Ca2+ signaling) as the molecular mechanism by which spatial differences of Vmem regulate organogenesis of the vertebrate brain, and suggest voltage modulation as a tractable strategy for intervention in certain classes of birth defects.