How ferroelectrics could replace silicon in computers
October 27, 2014
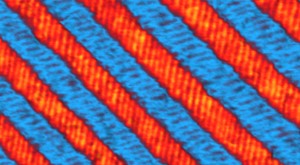
This herringbone pattern of nanoscale domains could enable faster switching in ferroelectric materials (credit: Ruijuan Xu and Lane W. Martin, UC Berkeley)
Ferroelectric materials — commonly used in RFID cards and video game memory — could become candidates for use in next-generation low-power computing and electronics, new research suggests.
Ferroelectric materials have spontaneous polarization as a result of small shifts of negative and positive charges within the material. The polarization can be reversed in response to an electric field, enabling the creation of a “0” or “1” data bit for memory applications.
So why haven’t ferroelectrics been used more widely, especially for processors? They’re too slow, according to the study authors.
“What we discovered was a fundamentally new and unexpected way for these ferroelectric materials to respond to applied electric fields,” said study principal investigator Lane Martin, UC Berkeley associate professor of materials science and engineering. “Our discovery opens up the possibility for faster switching and new control over novel, never-before-expected multi-state devices.”
Martin and other UC Berkeley researchers partnered with a team led by Andrew Rappe, University of Pennsylvania professor of chemistry and of materials science and engineering. They described their work the journal Nature Materials.
Advantages of ferroelectrics
Ferroelectric material holds a number of advantages over conventional semiconductors. Ferroelectrics are non-volatile (like flash memory), so they can remain in one polarized state or another without power. This ability makes them useful for transit cards, such as the Clipper cards used to pay fare in the Bay Area, and in certain memory cards for consumer electronics. If used in next-generation computers, ferroelectrics would enable the retention of information so that data would be there if electricity goes out.
The bits in a ferroelectric memory device depend on the position of metal atoms in the middle of six-pointed “cages” of oxygen atoms — atoms pointing up might represent a 1 and can be flipped down by an electric field to represent a 0. Those atoms, however, also could point to the left, right, front and back of those cages.
“Usually, ferroelectric bits of information are flipped from up to down, and this process can be too slow for modern computers,” said Rappe. “We predicted, and our colleagues found, experimentally, that sending the bits around the corner — flipping from up to left and then from left to down — can go much faster. This approach can enable a fundamentally new way to do computation with these materials.”
The researchers took a thin film of lead zirconate titanate, a common ferroelectric material, and found that by applying the electric field so that it wasn’t parallel to the up-down axis, they could change the polarization reorientation pathway. This opens up a faster, alternate route for the electrical charges to follow. Adjusting how the ferroelectric film was confined and stretched enhanced the effect, leading to at least a doubling of the operational speed for the system.
“These experiments showed that there’s an easy method of increasing the operational speed for ferroelectric materials,” said Martin. “We only looked at one material out of hundreds of options, so it’s very possible that we can get greater enhancements of speed by adjusting other variables, including film thickness.”
The National Science Foundation, the Department of Energy, the Office of Naval Research, and the Army Research Office helped support this research.
Abstract of Ferroelectric polarization reversal via successive ferroelastic transitions
Switchable polarization makes ferroelectrics a critical component in memories, actuators and electro-optic devices, and potential candidates for nanoelectronics. Although many studies of ferroelectric switching have been undertaken, much remains to be understood about switching in complex domain structures and in devices. In this work, a combination of thin-film epitaxy, macro- and nanoscale property and switching characterization, and molecular dynamics simulations are used to elucidate the nature of switching in PbZr0.2Ti0.8O3 thin films. Differences are demonstrated between (001)-/(101)- and (111)-oriented films, with the latter exhibiting complex, nanotwinned ferroelectric domain structures with high densities of 90° domain walls and considerably broadened switching characteristics. Molecular dynamics simulations predict both 180° (for (001)-/(101)-oriented films) and 90° multi-step switching (for (111)-oriented films) and these processes are subsequently observed in stroboscopic piezoresponse force microscopy. These results have implications for our understanding of ferroelectric switching and offer opportunities to change domain reversal speed.