How to print stronger, bigger, conductive 3-D graphene structures for tissue engineering
May 20, 2015
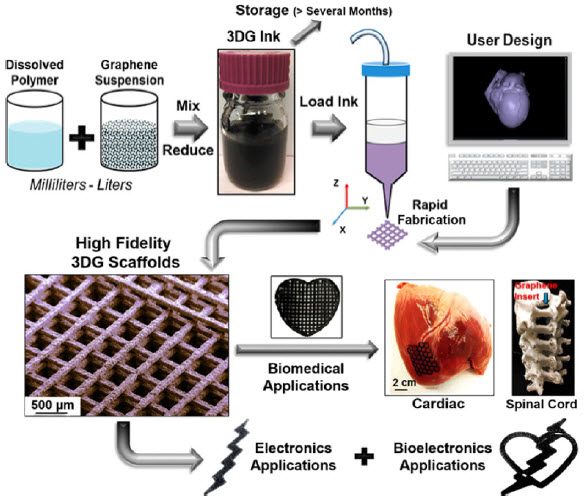
3D graphene inks are produced by simple combination and mixing an elastomer solution with graphene powder in a graded solvent, followed by volume reduction and thickening, a process that can be scaled up to many liters at once (credit: Adam E. Jakus et al./ACS Nano)
Northwestern University researchers have developed a way to print large, robust 3-D structures with graphene-based ink.
The new method could allow for using graphene-printed scaffolds for regenerative medicine and other medical and electronic applications.
“People have tried to print graphene before,” said Ramille Shah, assistant professor of materials science and engineering at the McCormick School of Engineering and of surgery in the Feinberg School of Medicine. “But it’s been a mostly polymer composite with graphene making up less than 20 percent of the volume.”
Adding higher volumes of graphene flakes to the mix in these ink systems typically results in printed structures too brittle and fragile to manipulate. At 60–70 percent graphene, the new ink preserves the material’s unique properties, including its electrical conductivity. And it’s flexible and robust enough to print robust macroscopic structures.
The secret: graphene nanoflakes are mixed with a biocompatible elastomer and fast-evaporating solvents.
“After the ink is extruded, one of the solvents in the system evaporates right away, causing the structure to solidify nearly instantly,” Shah explained. “The presence of the other solvents and the interaction with the specific polymer binder chosen also has a significant contribution to its resulting flexibility and properties. Because it holds its shape, we are able to build larger, well-defined objects.”
Could allow neurons to grow and communicate
Shah said her team populated one of the scaffolds with stem cells to surprising results. Not only did the cells survive; they divided, proliferated, and morphed into neuron-like cells.
The printed graphene structure is also flexible and strong enough to be easily sutured to existing tissues, so it could be used for biodegradable sensors and medical implants. Shah said the biocompatible elastomer and graphene’s electrical conductivity most likely contributed to the scaffold’s biological success.
“Cells conduct electricity inherently — especially neurons,” Shah said. “So if they’re on a substrate that can help conduct that signal, they’re able to communicate over wider distances.”
Supported by a Google Gift and a McCormick Research Catalyst Award, the research is described in the paper published in the April 2015 issue of ACS Nano.
Abstract of Three-Dimensional Printing of High-Content Graphene Scaffolds for Electronic and Biomedical Applications
The exceptional properties of graphene enable applications in electronics, optoelectronics, energy storage, and structural composites. Here we demonstrate a 3D printable graphene (3DG) composite consisting of majority graphene and minority polylactide-co-glycolide, a biocompatible elastomer, 3D-printed from a liquid ink. This ink can be utilized under ambient conditions via extrusion-based 3D printing to create graphene structures with features as small as 100 μm composed of as few as two layers (<300 μm thick object) or many hundreds of layers (>10 cm thick object). The resulting 3DG material is mechanically robust and flexible while retaining electrical conductivities greater than 800 S/m, an order of magnitude increase over previously reported 3D-printed carbon materials. In vitro experiments in simple growth medium, in the absence of neurogenic stimuli, reveal that 3DG supports human mesenchymal stem cell (hMSC) adhesion, viability, proliferation, and neurogenic differentiation with significant upregulation of glial and neuronal genes. This coincides with hMSCs adopting highly elongated morphologies with features similar to axons and presynaptic terminals. In vivo experiments indicate that 3DG has promising biocompatibility over the course of at least 30 days. Surgical tests using a human cadaver nerve model also illustrate that 3DG has exceptional handling characteristics and can be intraoperatively manipulated and applied to fine surgical procedures. With this unique set of properties, combined with ease of fabrication, 3DG could be applied toward the design and fabrication of a wide range of functional electronic, biological, and bioelectronic medical and nonmedical devices.