How to replace a $100,000 microscope with a Kinect game device
November 23, 2015
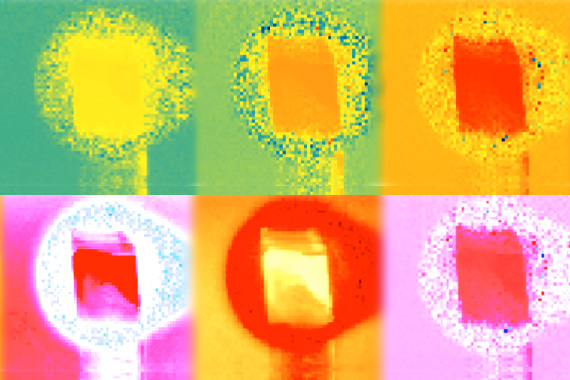
MIT researchers have developed a new biomedical imaging system that harnesses an off-the-shelf depth sensor such as Microsoft’s Kinect. The coloration of these images depicts the phase information contained in six of the 50 light frequencies the system analyzes. (credit: Ayush Bhandari, Christopher Barsi, and Ramesh Raskar/Optica)
MIT researchers have developed a new version of “fluorescence lifetime imaging microscopy” that could ultimately replace a $100,000 microscope with commercially available depth sensors that cost just hundreds of dollars.* The new system has applications in DNA sequencing and tumor detection, among other things.
The idea is to replace the electronic and optical precision of a large, expensive microscope with a low-cost device such as the Microsoft Kinect, using sophisticated mathematical modeling.
Imaging by measuring “time of flight”
The new method depends on fluorescence — the property of materials known as fluorophores (dyes added to a sample being studied) to absorb light and then fluoresce (re-emit it) later. For a given fluorophore, interactions with other chemicals (the sample being studied) will shorten the interval between absorption and re-emission of light in a predictable way. Measuring that interval — the “lifetime” of the fluorescence — in a biological sample treated with a fluorescent dye can reveal information about the sample’s chemical composition.
The MIT researchers reported the new work in an open-access paper in the Nov. 20 issue of the journal Optica. The research is headed by associate professor of media arts and sciences Ramesh Raskar and Christopher Barsi, a former research scientist in Raskar’s group who now teaches physics at the Commonwealth School in Boston.
Technical details
A traditional fluorescence lifetime imaging system emits a burst of light, much of which is absorbed by the sample, and then measures time-of-flight (how long it takes for returning light to strike an array of detectors). To make the measurement as precise as possible, the light bursts are extremely short — for biomedical imaging, in the nanosecond range.
Commercial depth sensors like the Kinect use light bursts that last tens of nanoseconds — too coarse-grained for fluorescence lifetime imaging. The Media Lab researchers, however, extract additional information from the Kinect light signal by subjecting it to a Fourier transform**.
The optical signal returning from the sample is represented as the sum of 50 different frequencies. Some of those frequencies are higher than that of the signal itself, which is how they are able to recover information about fluorescence lifetimes shorter than the duration of the emitted burst of light.
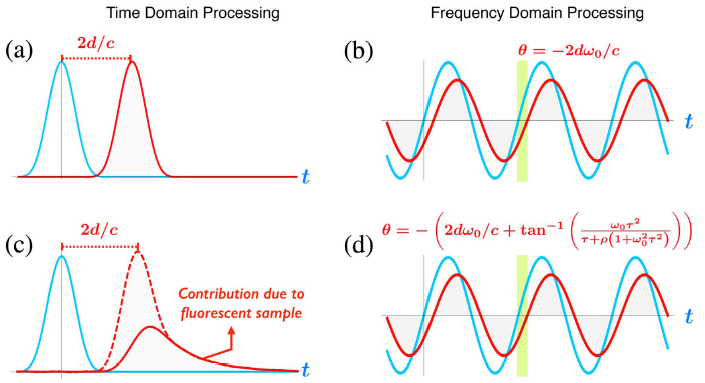
Time domain and frequency domain time-of-flight methods for fluorescence-lifetime imaging. (a) Probing and reflected signal for time domain time-of-flight. The time delay is proportional to distance d. (b) Probing and reflected signal for frequency domain time-of-flight. The phase is proportional to distance d and modulation frequency ω0. (c) Probing and reflected signal for time domain time-of-flight. The time delay is proportional to distance d and the waveform shape is linked with lifetime t. (d) Probing and reflected signal for frequency domain time-of-flight. The phase is proportional to distance d, modulation frequency ω0, and lifetime t. (credit: A. Bhandari et al./Optica)
For each of those 50 frequencies, the researchers measure the difference in phase (time delay) between the emitted signal and the returning signal. In fluorescence imaging, this phase shift also carries information about the fluorescence lifetime.
There is also reflected light from a sample. The MIT researchers’ system takes the measurements of both reflected and re-emitted light and calculates the distance between the emitter and the sample (no distance calibration required).
* Disclaimer by MIT Media Lab: “The depth sensors that the researchers used in their experiments — the Kinect and others — had arrays of roughly 20,000 light detectors each, and the most accurate results came when the detector was 2.5 meters away from the biological sample. That setup doesn’t afford the image resolution that existing fluorescence lifetime imaging microscopes do. But while denser arrays of detectors and optics that better control the emission and gathering of light would inflate the cost of the researchers’ system beyond the $100 that a Kinect costs, it still shouldn’t be nearly as expensive as current fluorescence lifetime imaging systems.”
** A Fourier transform is a technique for breaking signals — optical, electrical, or acoustical — into their constituent frequencies. A given signal, no matter how irregular, can be represented as the weighted sum of signals at many different frequencies, each of them perfectly regular.
Abstract of Blind and reference-free fluorescence lifetime estimation via consumer time-of-flight sensors
Fluorescence lifetime imaging (FLI) is a popular method for extracting useful information that is otherwise unavailable from a conventional intensity image. Usually, however, it requires expensive equipment, is often limited to either distinctly frequency- or time-domain modalities, and demands calibration measurements and precise knowledge of the illumination signal. Here, we present a generalized time-based, cost-effective method for estimating lifetimes by repurposing a consumer-grade time-of-flight sensor. By developing mathematical theory that unifies time- and frequency-domain approaches, we can interpret a time-based signal as a combination of multiple frequency measurements. We show that we can estimate lifetimes without knowledge of the illumination signal and without any calibration. We experimentally demonstrate this blind, reference-free method using a quantum dot solution and discuss the method’s implementation in FLI applications.