Neural prosthesis restores normal behavior after brain injury
December 11, 2013
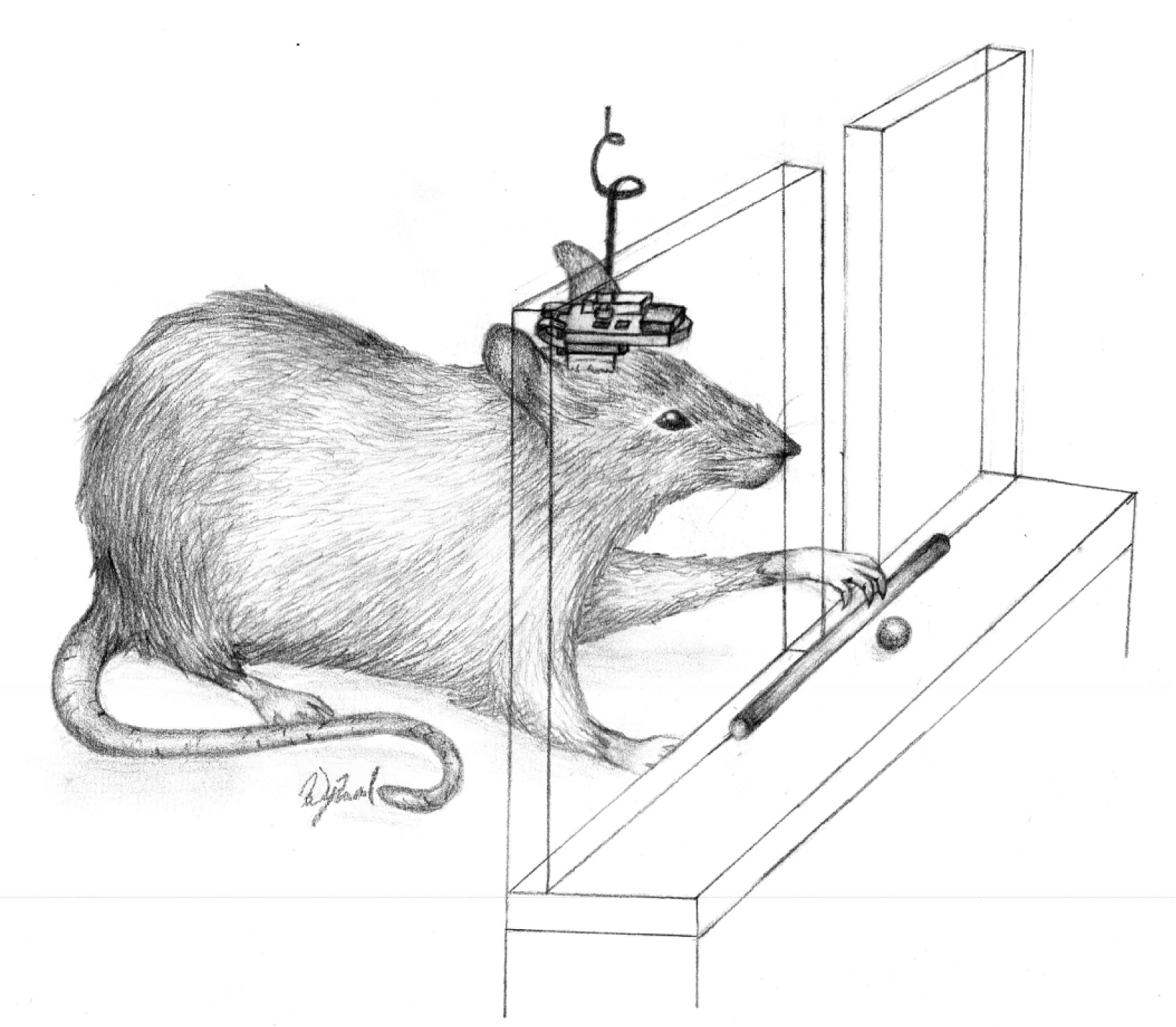
A rat retrieving a food pellet with a BMBI attached to the skull (credit: Case Western Reserve University)
Scientists from Case Western Reserve University and University of Kansas Medical Center have restored behavior — in this case, the ability to reach through a narrow opening and grasp food — using a neural prosthesis in a brain-injured rat.
Ultimately, the team hopes to develop a device that rapidly and substantially improves function after brain injury in humans.
There is no such commercial treatment for the 1.5 million Americans, including soldiers in Afghanistan and Iraq, who suffer traumatic brain injuries (TBI), or the nearly 800,000 stroke victims who suffer weakness or paralysis in the U.S. annually.
A ‘brain-machine-brain interface’
The prosthesis, called a brain-machine-brain interface (BMBI), is a closed-loop microelectronic system. It records signals from one part of the brain, processes them in real time, and then bridges the injury by stimulating a second part of the brain that had lost connectivity.
Their work is published online this week in the science journal Proceedings of the National Academy of Sciences (open access).
Is it possible to induce recovery from TBI if you use the device to couple activity from one part of the brain to another?
To find out, the researchers tested the BMBI prosthesis in a rat model of brain injury in the laboratory of Randolph J. Nudo, professor of molecular and integrative physiology at the University of Kansas. Nudo mapped the rat’s brain and developed the model in which anterior and posterior parts of the brain that control the rat’s forelimbs are disconnected.
How does it work?
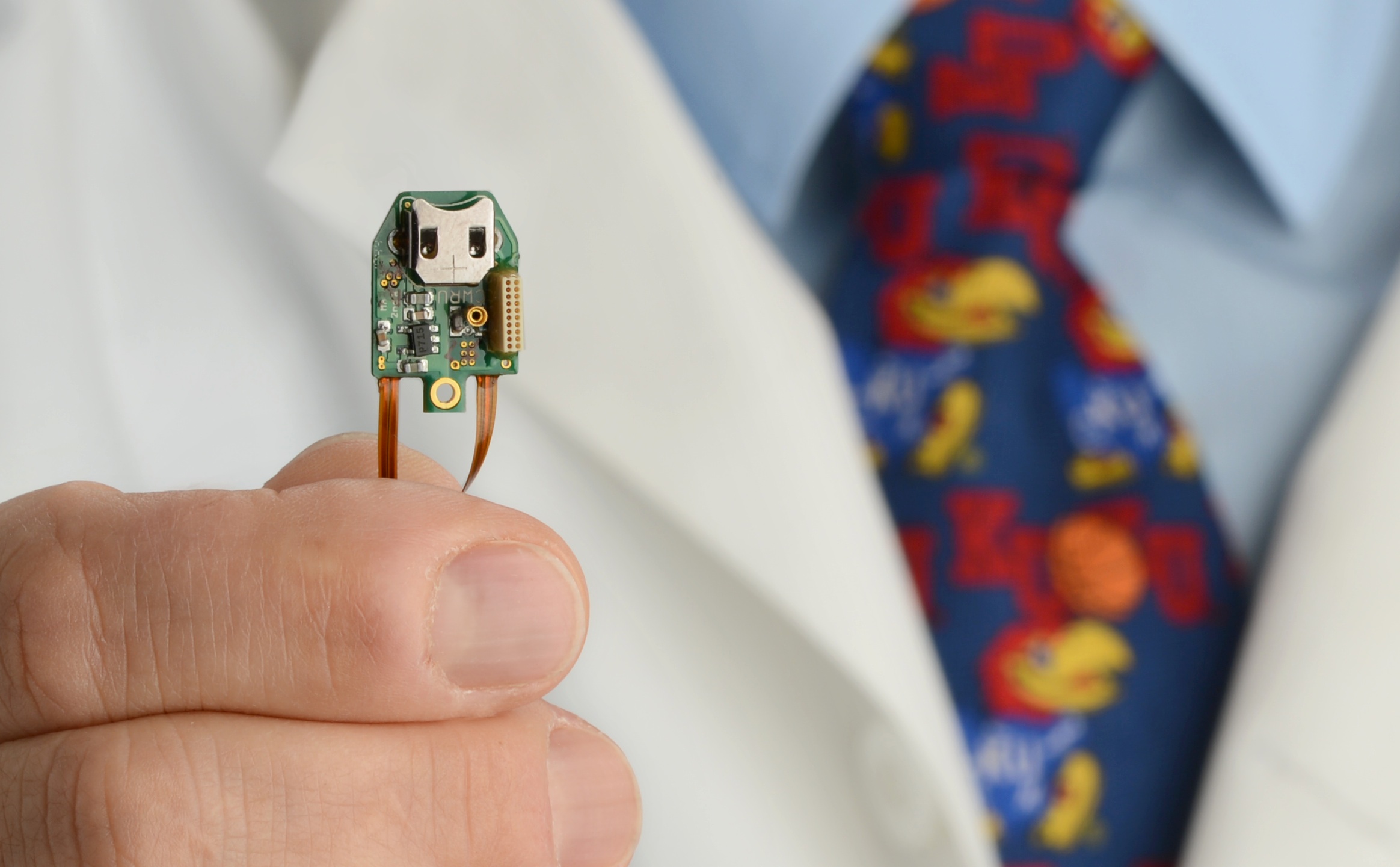
Brain-repair microdevice (credit: Case Western Reserve University)
Atop each animal’s head, the brain-machine-brain interface is a microchip on a circuit board smaller than a quarter, connected to microelectrodes implanted in the two brain regions.
Pedram Mohseni, professor of electrical engineering and computer science at Case Western Reserve, who built the brain prosthesis, described how it works:
- The device amplifies neural action potentials (signals) produced by the neurons in the anterior (front) of the brain.
- An algorithm separates these signals (recorded as brain spike activity) from noise and other artifacts.
- With each spike detected, the microchip sends a pulse of electric current to stimulate neurons in the posterior part of the brain, artificially connecting the two brain regions.
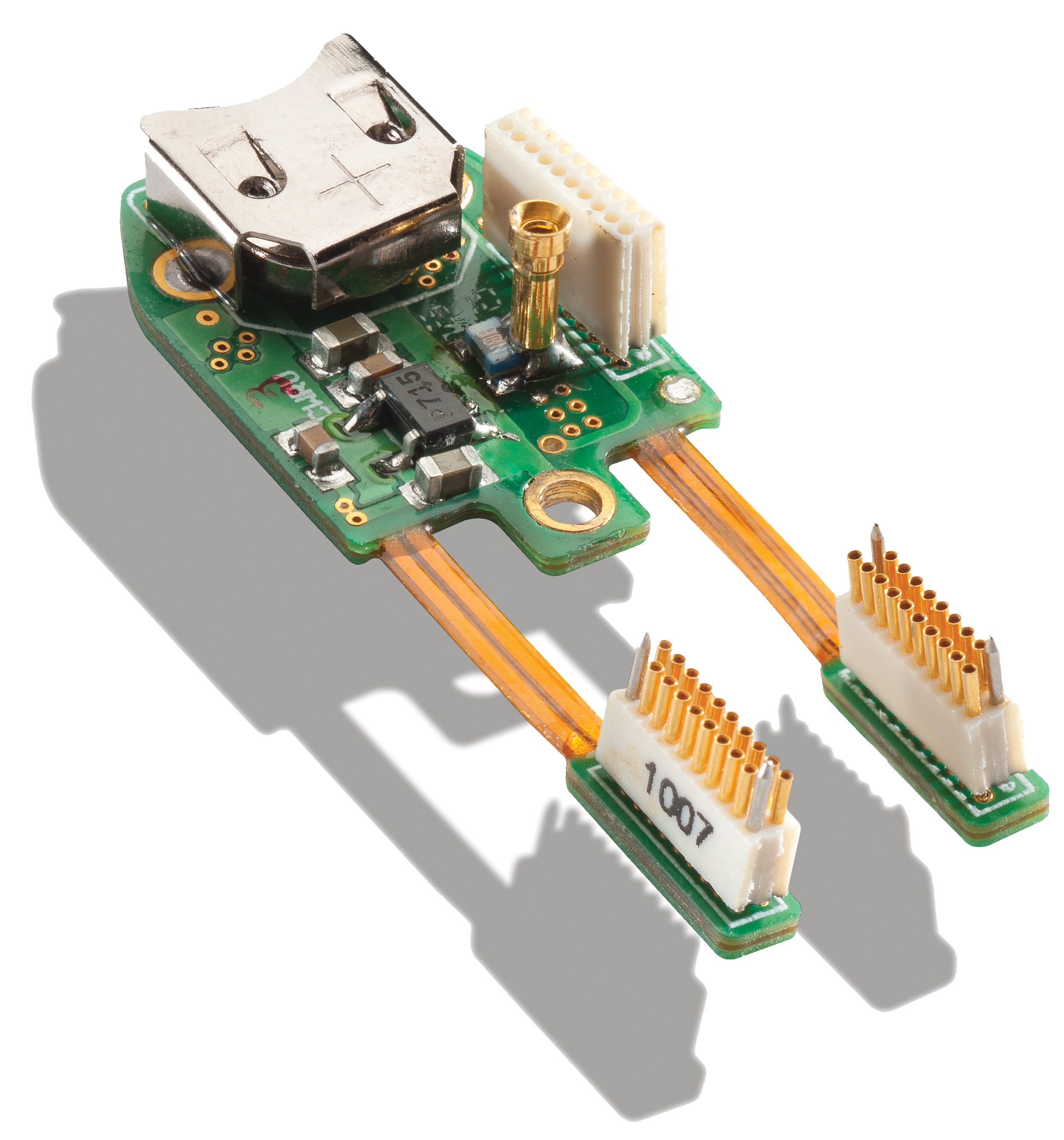
The neural prosthesis (credit: Case Western Reserve University)
Two weeks after the prosthesis had been implanted and run continuously, the rat models using the full closed-loop system had recovered nearly all function lost due to injury, successfully retrieving a food pellet close to 70 percent of the time, or as well as normal uninjured rats.
Rat models that received random stimuli from the device retrieved less than half the pellets and those that received no stimuli retrieved about a quarter of them.
“A question still to be answered is: must the implant be left in place for life? Or can it be removed after two months or six months, if and when new connections have been formed in the brain?”
Brain studies have shown that during periods of growth, neurons that regularly communicate with each other develop and solidify connections.
Mohseni and Nudo said they need more systematic studies to determine what happens in the brain that leads to such restoration of function. They also want to determine if there is an optimal time window after injury in which they must implant the device in order to restore function.
So when can we expect to see this available to humans?
“This technology could have direct clinical application for restoring neural communication in the brain, and thus, for restoring function,” Nudo explained to KurzweilAI in an email interview. “Initial clinical targets will include focal stroke or traumatic brain injury (TBI) for motor impairments.
“However, this technology may be effective for a variety of neurological syndromes that are thought to be related to disruption of cortical communication, such as disconnection syndromes. This could include applications for cognitive and psychiatric disorders, as well. From a practical standpoint, it is theoretically possible that the target locations for the technology could be identified using modern neuroimaging techniques, such as diffusion tensor imaging, or resting state connectivity.
“The innovation can be commercialized immediately, from the standpoint of initiating further development of a human device, navigating the FDA process for submitting an investigational device exemption (IDE), and identifying the most appropriate clinical population for first-in-human studies,” Nudo said. “Realistically, the pace of this process is constrained only by available financial resources. But, first-in-human studies could potentially take place in the 5–10 year time frame.”
And how does this development compare to other brain protheses?
“This brain-machine-brain interface (BMBI) neuroprosthesis is among the first examples of closed-loop neural interfaces that combine neural recording, neural signal processing and neuromodulation functions for real-time, bidirectional interfacing with the central nervous system, and is the first such example that is applied to facilitate rapid and substantial recovery from TBI,” said Mohseni.
“Other technologies that have been used to modulate brain activity in neurological conditions have been open-loop approaches (epidural stimulation, transcranial magnetic stimulation, transcranial direct-current stimulation). In addition, open-loop modulation is typically paired with rehabilitative therapy.
“In clinical trials, the rehabilitative therapy is often found to be the main factor, and not the neuromodulation technology. With this new closed-loop microdevice, at least in the rat model of TBI, no rehabilitation was required. The ‘training’ was induced in the neural pathway that was the target of the microdevice.”
Co-authors of the study include David J. Guggenmos, Scott Barbay and Caleb Dunham, of the department of molecular and integrative physiology, and Jonathan D. Mahnken, of the department of biostatistics, at Kansas University; and Meysam Azin, of QualComm, San Diego, who at the time was a Ph.D. student in Mohseni’s lab at Case Western Reserve’s electrical engineering and computer science department.
The work was funded by grants from the U.S. Army Medical Research and Materiel Command and the American Heart Association. The Advanced Platform Technology (APT) Center, a Veterans Affairs Research Center of Excellence in Cleveland supported the fabrication costs for the microchip in the prosthesis.
Abstract of Proceedings of the National Academy of Sciences paper
Neural interface systems are becoming increasingly more feasible for brain repair strategies. This paper tests the hypothesis that recovery after brain injury can be facilitated by a neural prosthesis serving as a communication link between distant locations in the cerebral cortex. The primary motor area in the cerebral cortex was injured in a rat model of focal brain injury, disrupting communication between motor and somatosensory areas and resulting in impaired reaching and grasping abilities. After implantation of microelectrodes in cerebral cortex, a neural prosthesis discriminated action potentials (spikes) in premotor cortex that triggered electrical stimulation in somatosensory cortex continuously over subsequent weeks. Within 1 wk, while receiving spike-triggered stimulation, rats showed substantially improved reaching and grasping functions that were indistinguishable from prelesion levels by 2 wk. Post hoc analysis of the spikes evoked by the stimulation provides compelling evidence that the neural prosthesis enhanced functional connectivity between the two target areas. This proof-of-concept study demonstrates that neural interface systems can be used effectively to bridge damaged neural pathways functionally and promote recovery after brain injury.