New magnetism-control method could lead to ultrafast, energy-efficient computer memory
November 3, 2017
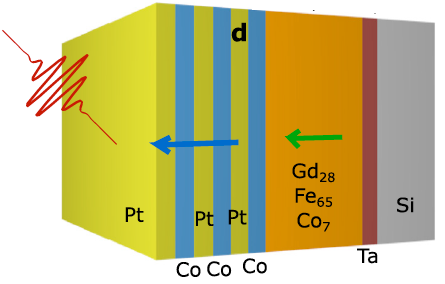
A cobalt layer on top of a gadolinium-iron alloy allows for switching memory with a single laser pulse in just 7 picoseconds. The discovery may lead to a computing processor with high-speed, non-volatile memory right on the chip. (credit: Jon Gorchon et al./Applied Physics Letters)
Researchers at UC Berkeley and UC Riverside have developed an ultrafast new method for electrically controlling magnetism in certain metals — a breakthrough that could lead to more energy-efficient computer memory and processing technologies.
“The development of a non-volatile memory that is as fast as charge-based random-access memories could dramatically improve performance and energy efficiency of computing devices,” says Berkeley electrical engineering and computer sciences (EECS) professor Jeffrey Bokor, coauthor of a paper on the research in the open-access journal Science Advances. “That motivated us to look for new ways to control magnetism in materials at much higher speeds than in today’s MRAM.”
Background: RAM vs. MRAM memory
Computers use different kinds of memory technologies to store data. Long-term memory, typically a hard disk or flash drive, needs to be dense in order to store as much data as possible but is slow. The central processing unit (CPU) — the hardware that enables computers to compute — requires fast memory to keep up with the CPU’s calculations, so the memory is only used for short-term storage of information (while operations are executed).
Random access memory (RAM) is one example of such short-term memory. Most current RAM technologies are based on charge (electron) retention, and can be written at rates of billions of bits per second (bits/nanosecond). The downside of these charge-based technologies is that they are volatile, requiring constant power or else they will lose the data.
In recent years, “spintronics” magnetic alternatives to RAM, known as Magnetic Random Access Memory (MRAM), have reached the market. The advantage of using magnets is that they retain information even when memory and CPU are powered off, allowing for energy savings. But that efficiency comes at the expense of speed, which is on the order of hundreds of picoseconds to write a single bit of information. (For comparison, silicon field-effect transistors have switching delays less than 5 picoseconds.)
The researchers found a magnetic alloy made up of gadolinium and iron that could accomplish those higher speeds — switching the direction of the magnetism with a series of electrical pulses of about 10 picoseconds (one picosecond is 1,000 times shorter than one nanosecond) — more than 10 times faster than MRAM.*
A faster version, using an energy-efficient optical pulse
In a second study, published in Applied Physics Letters, the researchers were able to further improve the performance by stacking a single-element magnetic metal such as cobalt on top of the gadolinium-iron alloy, allowing for switching with a single laser pulse in just 7 picoseconds. As a single pulse, it was also more energy-efficient. The result was a computing processor with high-speed, non-volatile memory right on the chip, functionally similar to an IBM Research “in-memory” computing architecture profiled in a recent KurzweilAI article.
“Together, these two discoveries provide a route toward ultrafast magnetic memories that enable a new generation of high-performance, low-power computing processors with high-speed, non-volatile memories right on chip,” Bokor says.
The research was supported by grants from the National Science Foundation and the U.S. Department of Energy.
* The electrical pulse temporarily increases the energy of the iron atom’s electrons, causing the magnetism in the iron and gadolinium atoms to exert torque on one another, and eventually leads to a reorientation of the metal’s magnetic poles. It’s a completely new way of using electrical currents to control magnets, according to the researchers.
Abstract of Ultrafast magnetization reversal by picosecond electrical pulses
The field of spintronics involves the study of both spin and charge transport in solid-state devices. Ultrafast magnetism involves the use of femtosecond laser pulses to manipulate magnetic order on subpicosecond time scales. We unite these phenomena by using picosecond charge current pulses to rapidly excite conduction electrons in magnetic metals. We observe deterministic, repeatable ultrafast reversal of the magnetization of a GdFeCo thin film with a single sub–10-ps electrical pulse. The magnetization reverses in ~10 ps, which is more than one order of magnitude faster than any other electrically controlled magnetic switching, and demonstrates a fundamentally new electrical switching mechanism that does not require spin-polarized currents or spin-transfer/orbit torques. The energy density required for switching is low, projecting to only 4 fJ needed to switch a (20 nm)3 cell. This discovery introduces a new field of research into ultrafast charge current–driven spintronic phenomena and devices.
Abstract of Single shot ultrafast all optical magnetization switching of ferromagnetic Co/Pt multilayers
A single femtosecond optical pulse can fully reverse the magnetization of a film within picoseconds. Such fast operation hugely increases the range of application of magnetic devices. However, so far, this type of ultrafast switching has been restricted to ferrimagnetic GdFeCo
films. In contrast, all optical switching of ferromagnetic films require multiple pulses, thereby being slower and less energy efficient. Here, we demonstrate magnetization switching induced by a single laser pulse in various ferromagnetic Co/Pt multilayers grown on GdFeCo, by exploiting
the exchange coupling between the two magnetic films. Table-top depth-sensitive time-resolved magneto-optical experiments show that the Co/Pt magnetization switches within 7 ps. This coupling approach will allow ultrafast control of a variety of magnetic films, which is critical for
applications.