round-up | Five important biomedical technology breakthroughs
March 30, 2018
Printing your own bioprinter
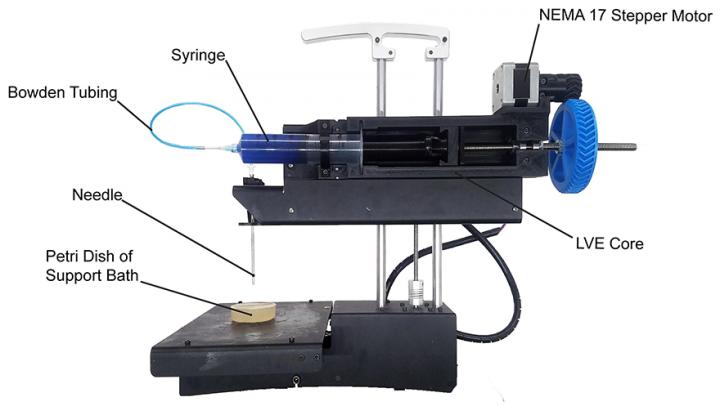
PrintrBot Simple Metal modified with the LVE for FRESH printing. (credit: Adam Feinberg/HardwareX)
Now you can build your own low-cost 3-D bioprinter by modifying a standard commercial desktop 3-D printer for under $500 — thanks to an open-source “LVE 3-D” design developed by Carnegie Mellon University (CMU) researchers. CMU provides detailed instructional videos.
You can print artificial human tissue scaffolds on a larger scale (entire human heart) and at higher resolution and quality, the researchers say. Most 3-D bioprinters start between $10K and $20K, and commercial 3D printers cost up to $200,000 and are typically proprietary machines, closed source, and difficult to modify.
AI-enhanced medical imaging technique reduces radiation doses, MRI times
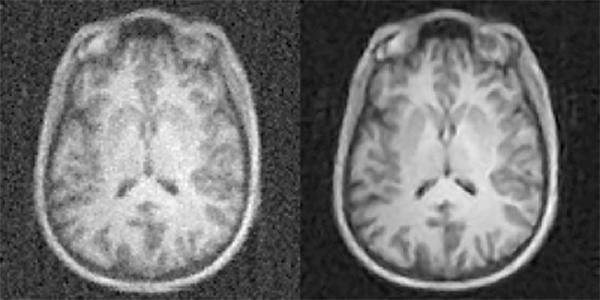
AUTOMAP yields higher-quality images medical images from less data, reducing radiation doses for CT and PET and shortening scan times for MRI. Shown here: MRI images reconstructed from the same data with conventional approaches (left) and AUTOMAP (right). (credit: Athinoula A. Martinos Center for Biomedical Imaging, Massachusetts General Hospital)
Massachusetts General Hospital (MGH) researchers have developed a machine-learning-based technique that enables clinicians to acquire higher-quality images without the increased radiation dose — from acquiring additional data from computed tomography (CT) or positron emission tomography (PET) — or the uncomfortably long scan times needed for magnetic resonance imaging (MRI).
The new AUTOMAP (automated transform by manifold approximation) deep-learning technique avoids radiologists having to tweak manual settings to overcome imperfections in raw data.
The technique could also help radiologists make real-time decisions about imaging protocols while the patient is in the scanner (image reconstruction time is just tens of milliseconds), thanks to AI algorithms running on graphical processing units (GPUs). — MGH Athinoula A. Martinos Center for Biomedical Imaging, Nature.
“Nanotweezers” trap cells and nanoparticles with a laser beam
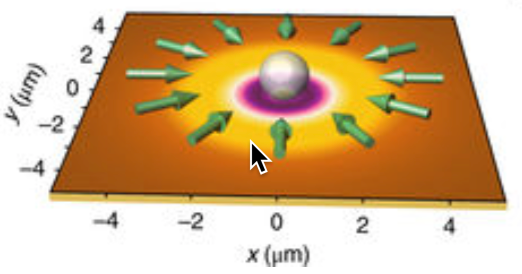
Trapping a nanoparticle with laser beams (credit: Linhan Lin et al./Nature Photonics)
A new tool called “opto-thermoelectric nanotweezers” (OTENT) allows bioscientists to use light to manipulate biological cells and molecules at single-molecule resolution. The goal is to make nanomedicine discoveries for early disease diagnosis.
By optically heating a plasmonic substrate, a light-directed thermoelectric field is generated by spatial separation of dissolved ions within the heating laser spot — allowing for manipulating nanoparticles over a wide range of materials, sizes and shapes. — University of Texas at Austin, Nature Photonics.
CMU Associate Professor Adam Feinberg says his lab “aims to produce open-source biomedical research that other researchers can expand upon … to seed innovation widely [and] encourage the rapid development of biomedical technologies to save lives.”— CMU, video, HardwareX (open-access)
Nanometer-scale MRI opens the door to viewing virus nanoparticles and proteins
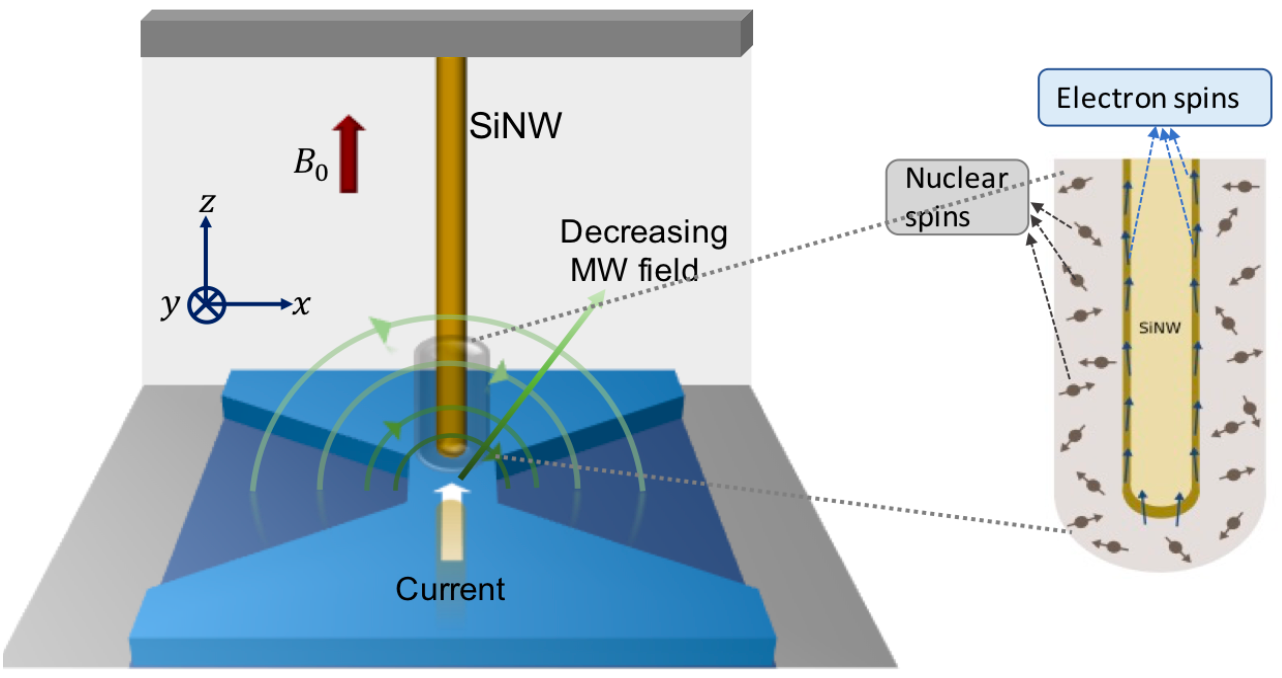
MRFM imaging device under microwave irradiation (inset: ultra-sensitive mechanical oscillator — a silicon nanowire, with sample coated at the tip (credit: R. Annabestani et al./Physical Review X)
A new technique allows for magnetic resonance imaging (MRI) at the unprecedented resolution of 2 nanometers — 10,000 times smaller than current millimeter resolution.
It promises to open the door to major advances in understanding virus particles, proteins that cause diseases like Parkinson’s and Alzheimer’s, and discovery of new materials, say researchers at the University of Waterloo Institute for Quantum Computing.
The breakthrough technique combines magnetic resonance force microscopy (MRFM) with the ability to precisely control atomic spins. “We now have unprecedented access to understanding complex biomolecules,” says Waterloo physicist Raffi Budakian. — Waterloo, Physical Review X, arXiv (open-access)
Skin-implantable biosensor relays real-time personal health data to a cell phone, lasts for years
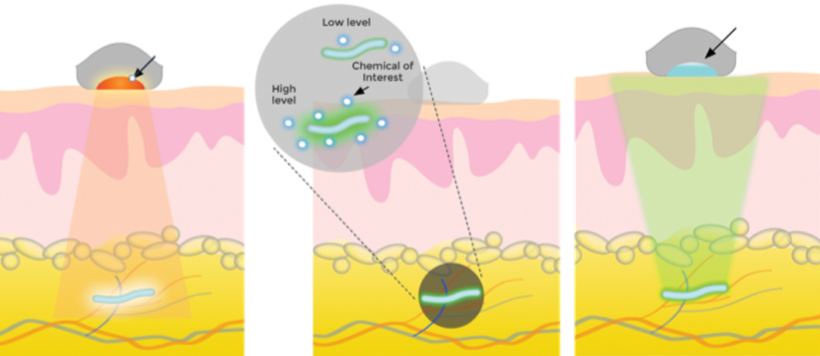
Left: Infrared light (arrow shows excitation light) causes a biosensor (blue) under the skin to fluoresce at a level determined by the chemical of interest (center). Right: A detector (arrow) receives and analyzes the signals from the biosensor and transmits data to a computer or phone. (credit: Profusa)
A technology for continuous monitoring of glucose, lactate, oxygen, carbon dioxide, and other molecules — using tiny biosensors that are placed under the skin with a single injection — has been developed by DARPA/NIH-supported Profusa. Using a flexible, biocompatible hydrogel fiber about 5mm long and 0.5mm wide, the biosensors can continuously measure body chemistries.
An external device sends light through the skin to the biosensor dye phosphor, which then emits light proportional to the concentration of the current analyte (such as glucose) of interest. A detector then wirelessly transmits the brightness measurement to a computer or cell phone to record the change. Data can be shared securely via digital networks with healthcare providers.
To date, the injected biosensors have functioned for as long as four years. For example, tracking the rise and fall of oxygen levels around muscle with these sensors produces an “oxygen signature” that may reveal a person’s fitness level.
Until now, local inflammation and scar tissue from the “foreign body response” (from a sensing electrode wire that penetrates the skin) has prevented development of in-body sensors capable of continuous, long-term monitoring of body chemistry. The Lumee Oxygen Platform, the first medical application of the biosensor technology, was approved in 2017 for sale in Europe, designed for patients undergoing treatment for chronic limb ischemia, avoiding amputations. — Profusa