Stem cells from teeth can make neuron-like cells and networks
May 6, 2014
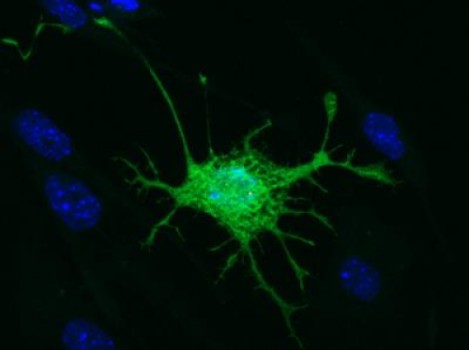
This is the distinct neuronal-like appearance of a mouse-derived dental pulp stem cell following the induction process (credit: Dr. Kylie Ellis, University of Adelaide)
University of Adelaide researchers have discovered that stem cells taken from teeth can grow to form complex networks of neuron-like cells, suggesting a possible therapy for stroke.
Although these cells haven’t developed into fully fledged neurons, researchers believe it’s just a matter of time and the right conditions for it to happen.
“Stem cells from teeth have great potential to grow into new brain or nerve cells, and this could potentially assist with treatments of brain disorders, such as stroke,” says Kylie Ellis, PhD, Commercial Development Manager with the University’s commercial arm, Adelaide Research & Innovation (ARI).
The stem cells expressed neuronal cytoplasmic proteins, neurotransmitter-specific markers, and functional voltage-gated L-type Ca2+ channels, but not spontaneous action potentials.
The results of her work have been published in the journal Stem Cell Research & Therapy (open access).
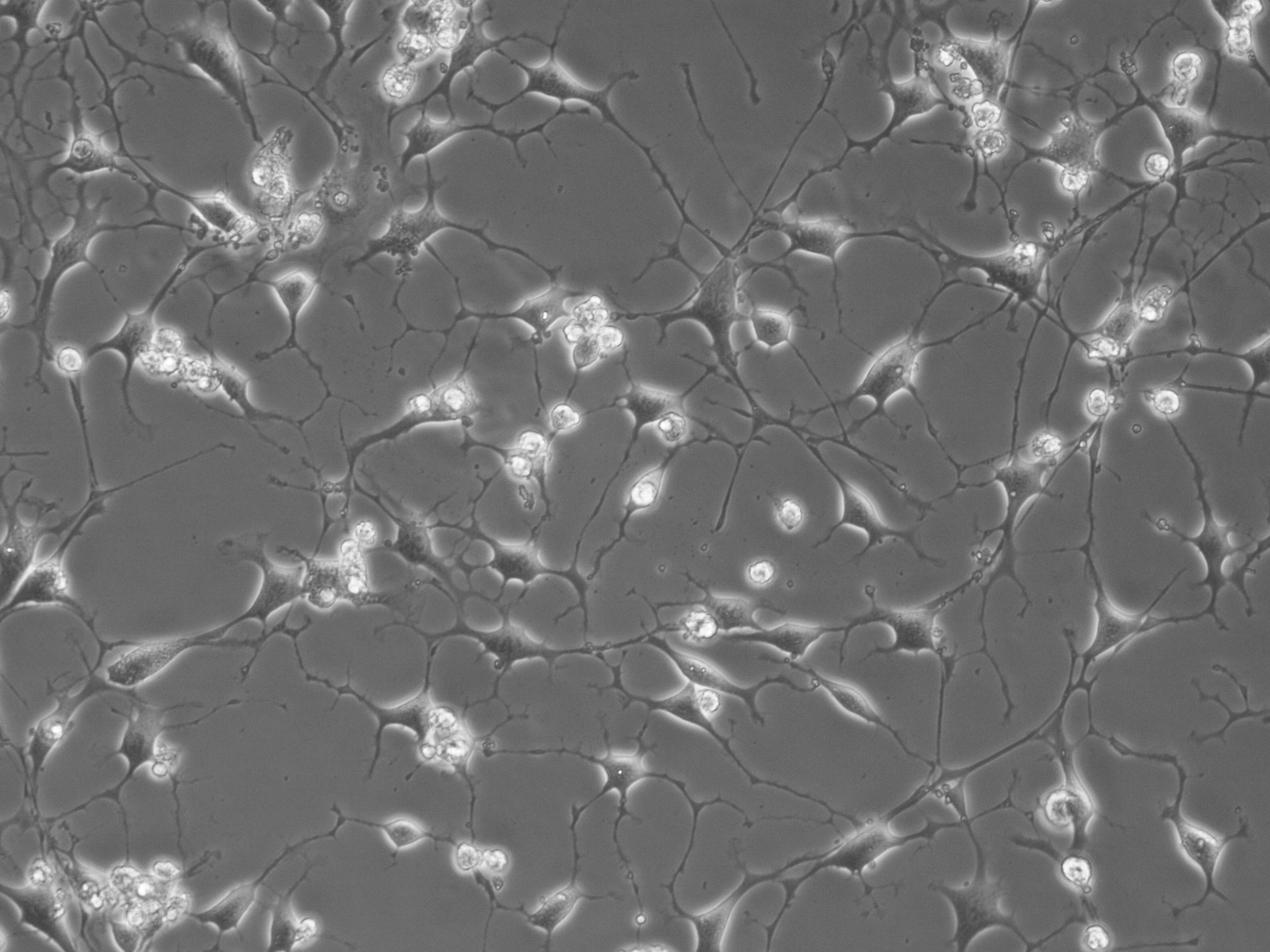
Mouse DPSC-derived brain-like cells forming complex network (credit: Dr. Kylie Ellis, University of Adelaide)
“The reality is, treatment options available to the thousands of stroke patients every year are limited,” Ellis says. “The primary drug treatment available must be administered within hours of a stroke and many people don’t have access within that timeframe, because they often can’t seek help for some time after the attack.
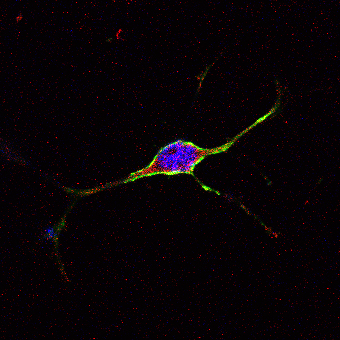
A mouse-derived dental pulp stem cell with a distinct neuronal-like appearance that is expressing brain-cell specific markers in green and red (credit: Dr. Kylie Ellis, University of Adelaide)
“Ultimately, we want to be able to use a patient’s own stem cells for tailor-made brain therapy that doesn’t have the host rejection issues commonly associated with cell-based therapies. Another advantage is that dental pulp stem cell therapy may provide a treatment option available months or even years after the stroke has occurred,” she says.
“We can do this by providing an environment for the cells that is as close to a normal brain environment as possible, so that instead of becoming cells for teeth they become brain cells,” Ellis says.
“What we developed wasn’t identical to normal neurons, but the new cells shared very similar properties to neurons. They also formed complex networks and communicated through simple electrical activity, like you might see between cells in the developing brain.”
Abstract of Stem Cell Research & Therapy paper
Introduction – Interest in the use of dental pulp stem cells (DPSC) to enhance neurological recovery following stroke and traumatic injury is increasing following successful pre-clinical studies. A murine model of autologous neural stem cell transplantation would be useful for further pre-clinical investigation of the underlying mechanisms. However, while human-derived DPSC have been well characterised, the neurogenic potential of murine DPSC (mDPSC) has been largely neglected. In this study we demonstrate neuronal differentiation of DPSC from murine incisors in vitro.
Methods – mDPSC were cultured under neuroinductive conditions and assessed for neuronal and glial markers and electrophysiological functional maturation.
Results – mDPSC developed a neuronal morphology and high expression of neural markers nestin, ßIII-tubulin and GFAP. Neurofilament M and S100 were found in lower abundance. Differentiated cells also expressed protein markers for cholinergic, GABAergic and glutaminergic neurons, indicating a mixture of central and peripheral nervous system cell types. Intracellular electrophysiological analysis revealed the presence of voltage-gated L-type Ca2+ channels in a majority of cells with neuronal morphology. No voltage-gated Na+ or K+ currents were found and the cultures did not support spontaneous action potentials. Neuronal-like networks expressed the gap junction protein, connexin 43 but this was not associated with dye coupling between adjacent cells after injection of the low-molecular weight tracers Lucifer yellow or Neurobiotin. This indicated that the connexin proteins were not forming traditional gap junction channels.
Conclusions – The data presented support the differentiation of mDPSC into immature neuronal-like networks.