Supermaterials improve solar collectors
January 21, 2015
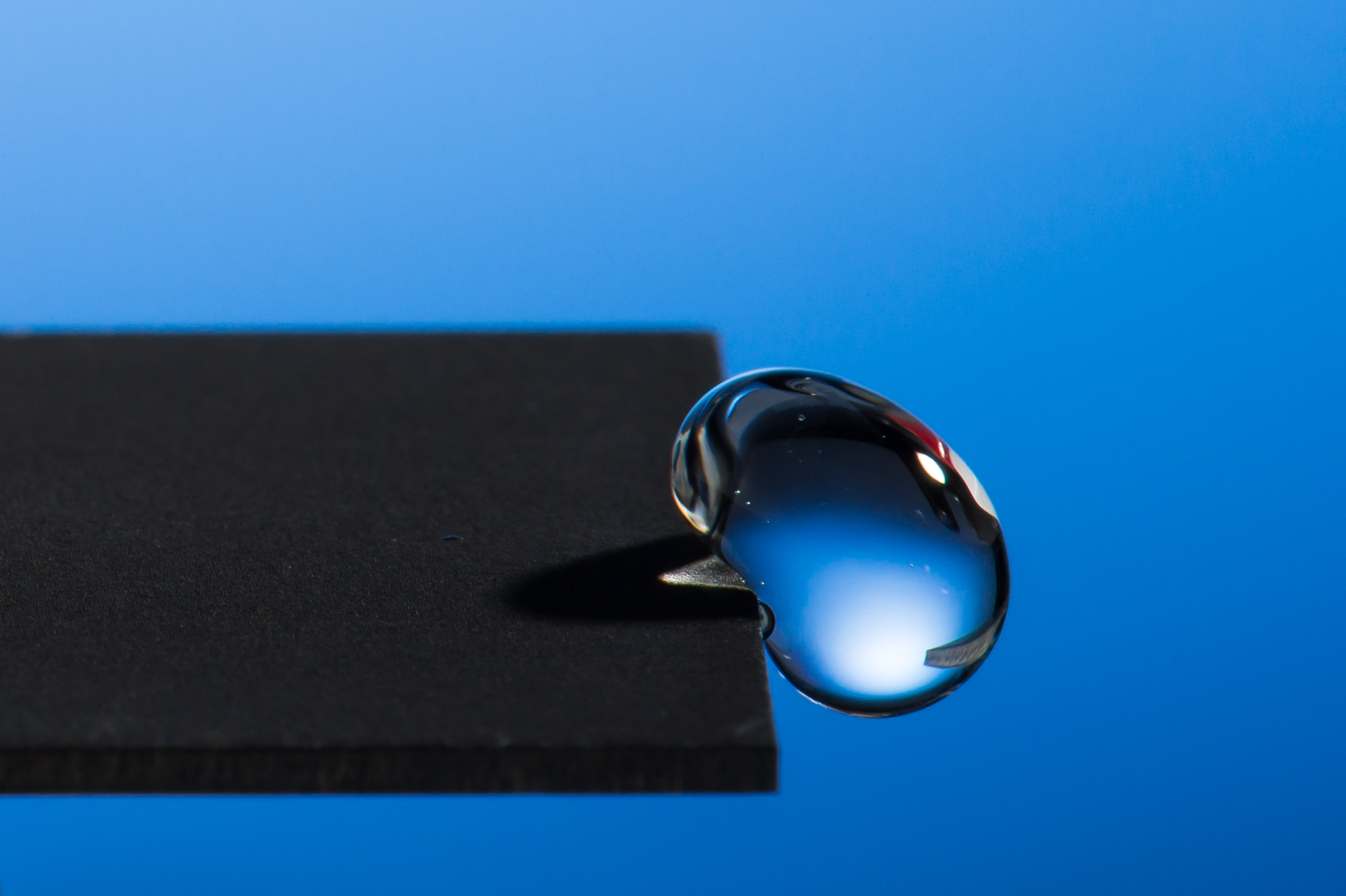
A new technique uses lasers to render materials hydrophobic, illustrated in this image of a water droplet falling off a treated sample (credit: J. Adam Fenster/University of Rochester)
By zapping ordinary metals with femtosecond laser pulses, researchers from the University of Rochester in New York have created extraordinary new surfaces that efficiently absorb light, repel water and clean themselves for use in durable, low-maintenance solar collectors and sensors, for example.
This is the first multifunctional metal surface created by lasers that is superhydrophobic (water repelling), self-cleaning, and highly absorptive,” said Chunlei Guo, a physicist at the Institute of Optics at the University of Rochester who made the new surfaces with University of Rochester researcher Anatoliy Vorobyev.
The researchers describe the laser-patterned surfaces in an open-access article published in the Journal of Applied Physics, from AIP Publishing.
Enhanced light absorption improves light collection in solar sensors, while superhydrophobicity will make a surface rust-resistant, anti-icing, and anti-biofouling, a self-cleaning to makes solar collectors robust and easier to maintain, Guo said.
The researchers created the surfaces by zapping platinum, titanium and brass samples with extremely short femtosecond laser pulses that lasted on the order of a millionth of a billionth of a second. “During its short burst the peak power of the laser pulse is equivalent to that of the entire power grid of North America,” Guo said.
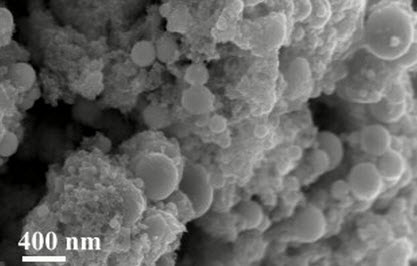
A femtosecond laser created detailed hierarchical structures in the metals, as shown in this SEM image of the platinum surface (credit: The Guo Lab/University of Rochester)
These extra-powerful laser pulses produced microgrooves, on top of which densely populated, lumpy nanostructures were formed. The structures alter the optical and wetting properties of the surfaces of the three metals, turning the normally shiny surfaces velvet black (very optically absorptive) and also making them water repellent.
Most commercially used hydrophobic and high optical absorption materials rely on chemical coatings that can degrade and peel off over time, said Guo. Because the nano- and microstructures created by the lasers are intrinsic to the metal, the properties they confer should not deteriorate, he said.
The hydrophobic properties of the laser-patterned metals also compare favorably with Teflon. “Many people think of Teflon as a hydrophobic surface, but if you want to get rid of water from a Teflon surface, you will have to tilt the surface to nearly 70 degrees before the water can slide off,” Guo said. “Our surface has a much stronger hydrophobicity and requires only a couple of degrees of tilt for water to slide off.”
The team has plans to work on creating multifunctional effects on other materials, such as semiconductors and dielectrics. The multifunctional effects should find a wide range of applications such as making better solar energy collectors.
University of Rochester | Using Lasers to Create Super-hydrophobic Materials
Reducing energy lost in reflections
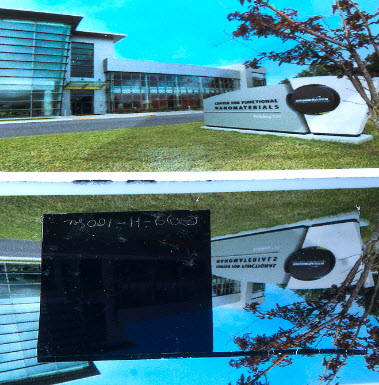
A closeup shows how the nanotextured square of silicon completely blocks reflection in the reflected image on a silicon wafer (bottom), compared with the surrounding silicon wafer (credit: BNL)
Another way to improve solar collectors is by reducing the amount of sunlight that bounces off the surface of solar cells.
That helps maximize the conversion of the sun’s rays to electricity, so manufacturers use coatings to cut down on reflections. Scientists at the U.S. Department of Energy’s Brookhaven National Laboratory have recently developed a method for etching a nanoscale texture onto the silicon material itself. That can create an antireflective surface that works as well as expensive state-of-the-art thin-film multilayer coatings.
Their method, described in the journal Nature Communications and submitted for patent protection, has potential for streamlining silicon solar cell production and reducing manufacturing costs. The approach may find additional applications in reducing glare from windows, providing radar camouflage for military equipment, and increasing the brightness of light-emitting diodes.
“For antireflection applications, the idea is to prevent light or radio waves from bouncing at interfaces between materials,” said physicist Charles Black, who led the research at Brookhaven Lab’s Center for Functional Nanomaterials (CFN), a DOE Office of Science User Facility.
Preventing reflections requires controlling an abrupt change in “refractive index,” a property that affects how waves such as light propagate through a material. This occurs at the interface where two materials with very different refractive indices meet, for example at the interface between air and silicon. Adding a coating with an intermediate refractive index at the interface eases the transition between materials and reduces the reflection, Black explained.
“The issue with using such coatings for solar cells,” he said, “is that we’d prefer to fully capture every color of the light spectrum within the device, and we’d like to capture the light irrespective of the direction it comes from. But each color of light couples best with a different antireflection coating, and each coating is optimized for light coming from a particular direction. So you deal with these issues by using multiple antireflection layers. We were interested in looking for a better way.”
Moth nanotechnology
For inspiration, the scientists turned to a well-known example of an antireflective surface in nature, the eyes of common moths. The surfaces of their compound eyes have textured patterns made of many tiny “posts,” each smaller than the wavelengths of light. This textured surface improves moths’ nighttime vision, and also prevents the “deer in the headlights” reflecting glow that might allow predators to detect them.
“We set out to recreate moth eye patterns in silicon at even smaller sizes using methods of nanotechnology,” said Atikur Rahman, a postdoctoral fellow working with Black at the CFN and first author of the study.
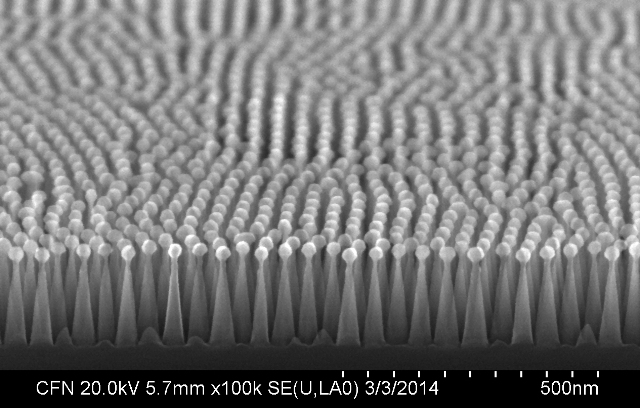
Details of the nanotextured antireflective surface as revealed by a scanning electron microscope at the Center for Functional Nanomaterials. The tiny posts, each smaller than the wavelengths of light, are reminiscent of the structure of moths’ eyes, an example of an antireflective surface found in nature. (Credit: BNL)
The scientists started by coating the top surface of a silicon solar cell with a polymer material called a “block copolymer,” which can be made to self-organize into an ordered surface pattern with dimensions measuring only tens of nanometers.
The self-assembled pattern served as a template for forming posts in the solar cell like those in the moth eye using a plasma of reactive gases—a technique commonly used in the manufacture of semiconductor electronic circuits.
The resulting surface nanotexture served to gradually change the refractive index to drastically cut down on reflection of many wavelengths of light simultaneously, regardless of the direction of light impinging on the solar cell.
“Adding these nanotextures turned the normally shiny silicon surface absolutely black,” Rahman said.
Solar cells textured in this way outperform those coated with a single antireflective film by about 20 percent, and bring light into the device as well as the best multi-layer-coatings used in the industry.
“We are working to understand whether there are economic advantages to assembling silicon solar cells using our method, compared to other, established processes in the industry,” Black said.
Nanoposts
One intriguing aspect of the study was that the scientists achieved the antireflective performance by creating nanoposts only half as tall as the required height predicted by a mathematical model describing the effect. Using a combination of computational modeling, electron microscopy, and surface science, the team deduced that a thin layer of silicon oxide similar to what typically forms when silicon is exposed to air seemed to be having an outsized effect.
“On a flat surface, this layer is so thin that its effect is minimal,” explained Matt Eisaman of Brookhaven’s Sustainable Energy Technologies Department and a professor at Stony Brook University. “But on the nanopatterned surface, with the thin oxide layer surrounding all sides of the nanotexture, the oxide can have a larger effect because it makes up a significant portion of the nanotextured material.”
Said Black, “This ‘hidden’ layer was the key to the extra boost in performance.”
The scientists are now interested in developing their self-assembly based method of nanotexture patterning for other materials, including glass and plastic, for antiglare windows and coatings for solar panels.
This research was supported by the DOE Office of Science.
Abstract of Multifunctional surfaces produced by femtosecond laser pulses
In this study, we create a multifunctional metal surface by producing a hierarchical nano/microstructure with femtosecond laser pulses. The multifunctional surface exhibits combined effects of dramatically enhanced broadband absorption, superhydrophobicity, and self-cleaning. The superhydrophobic effect is demonstrated by a falling water droplet repelled away from a structured surface with 30% of the droplet kinetic energy conserved, while the self-cleaning effect is shown by each water droplet taking away a significant amount of dust particles on the altered surface. The multifunctional surface is useful for light collection and water/dust repelling.
Abstract for Sub-50-nm self-assembled nanotextures for enhanced broadband antireflection in silicon solar cells
Materials providing broadband light antireflection have applications as highly transparent window coatings, military camouflage and coatings for efficiently coupling light into solar cells and out of light-emitting diodes. In this work, densely packed silicon nanotextures with feature sizes smaller than 50 nm enhance the broadband antireflection compared with that predicted by their geometry alone. A significant fraction of the nanotexture volume comprises a surface layer whose optical properties differ substantially from those of the bulk, providing the key to improved performance. The nanotexture reflectivity is quantitatively well modelled after accounting for both its profile and changes in refractive index at the surface.We employ block copolymer self-assembly for precise and tunable nanotexture design in the range of ~10–70nm across macroscopic solar cell areas. Implementing this efficient antireflection approach in crystalline silicon solar cells significantly betters the performance gain compared with an optimized, planar antireflection coating.