Future ‘lightwave’ computers could run 100,000 times faster
March 14, 2017
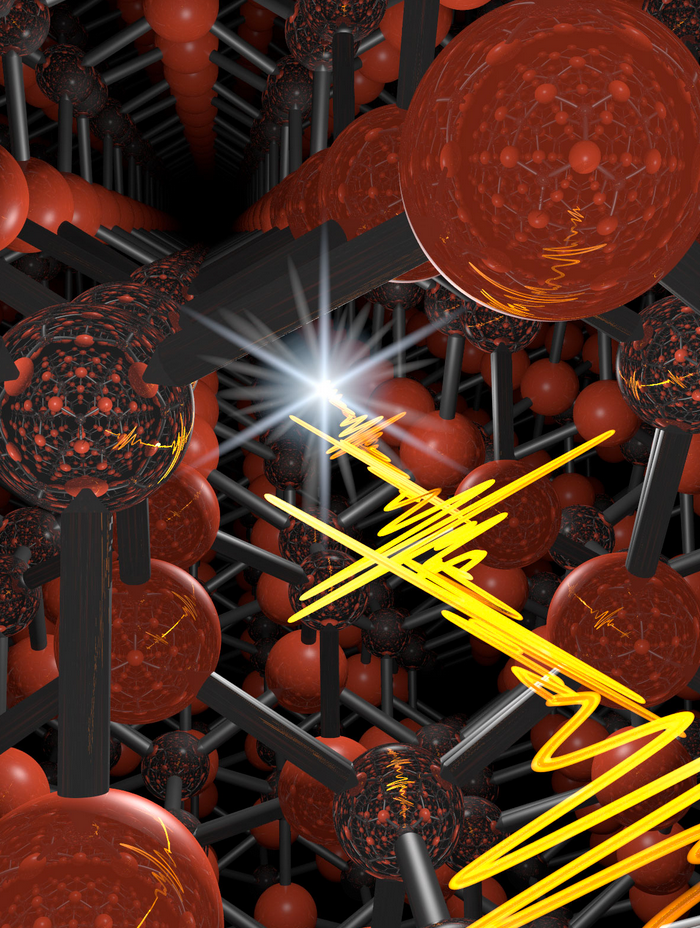
TeraHertz pulses in semiconductor crystal (credit: Fabian Langer, Regensburg University)
Using extremely short pulses of teraHertz (THz) radiation instead of electrical currents could lead to future computers that run ten to 100,000 times faster than today’s state-of-the-art electronics, according to an international team of researchers, writing in the journal Nature Photonics.
In a conventional computer, electrons moving through a semiconductor occasionally run into other electrons, releasing energy in the form of heat and slowing them down. With the proposed “lightwave electronics” approach, electrons could be guided by ultrafast THz pulses (the part of the electromagnetic spectrum between microwaves and infrared light). That means the travel time can be so short that the electrons would be statistically unlikely to hit anything, according to senior author Rupert Huber, a professor of physics at the University of Regensburg who led the experiment.
In the experiment, the researchers shined THz pulses into a crystal of the semiconductor gallium selenide.* These pulses were ultra-short (less than 100 femtoseconds, or 100 quadrillionths of a second). Each pulse popped electrons in the semiconductor into a higher energy level — which meant that they were free to move around.
When the electrons emitted light as they came down from the higher energy level, they emitted much shorter pulses than the electromagnetic radiation going in — just a few femtoseconds long — quick enough to read and write information to electrons at ultra-high speed.
But first, researchers need to be able to control electrons in a semiconductor. This work takes a step toward this by mobilizing groups of electrons inside a semiconductor crystal.
Quantum computation
Because femtosecond pulses are fast enough to trap an electron between being put into an excited state and coming down from that state, they can potentially also be used for quantum computations, using electrons in excited states as qubits. The researchers managed to launch one electron simultaneously via two excitation pathways, which is not classically possible.
An electron is small enough that it behaves like a wave as well as a particle, and when it is in an excited state, its wavelength changes. Because the electron was in two excited states at once, those two waves interfered with one another and left a fingerprint in the femtosecond pulse that the electron emitted.
The research is funded by the European Research Council and the German Research Foundation.
Abstract of Symmetry-controlled temporal structure of high-harmonic carrier fields from a bulk crystal
High-harmonic (HH) generation in crystalline solids marks an exciting development, with potential applications in high-efficiency attosecond sources, all-optical bandstructure reconstruction and quasiparticle collisions. Although the spectral and temporal shape of the HH intensity has been described microscopically, the properties of the underlying HH carrier wave have remained elusive. Here, we analyse the train of HH waveforms generated in a crystalline solid by consecutive half cycles of the same driving pulse. Extending the concept of frequency combs to optical clock rates, we show how the polarization and carrier-envelope phase (CEP) of HH pulses can be controlled by the crystal symmetry. For certain crystal directions, we can separate two orthogonally polarized HH combs mutually offset by the driving frequency to form a comb of even and odd harmonic orders. The corresponding CEP of successive pulses is constant or offset by π, depending on the polarization. In the context of a quantum description of solids, we identify novel capabilities for polarization- and phase-shaping of HH waveforms that cannot be accessed with gaseous sources.