Using lasers and carbon nanotubes to look inside living brains
August 7, 2014
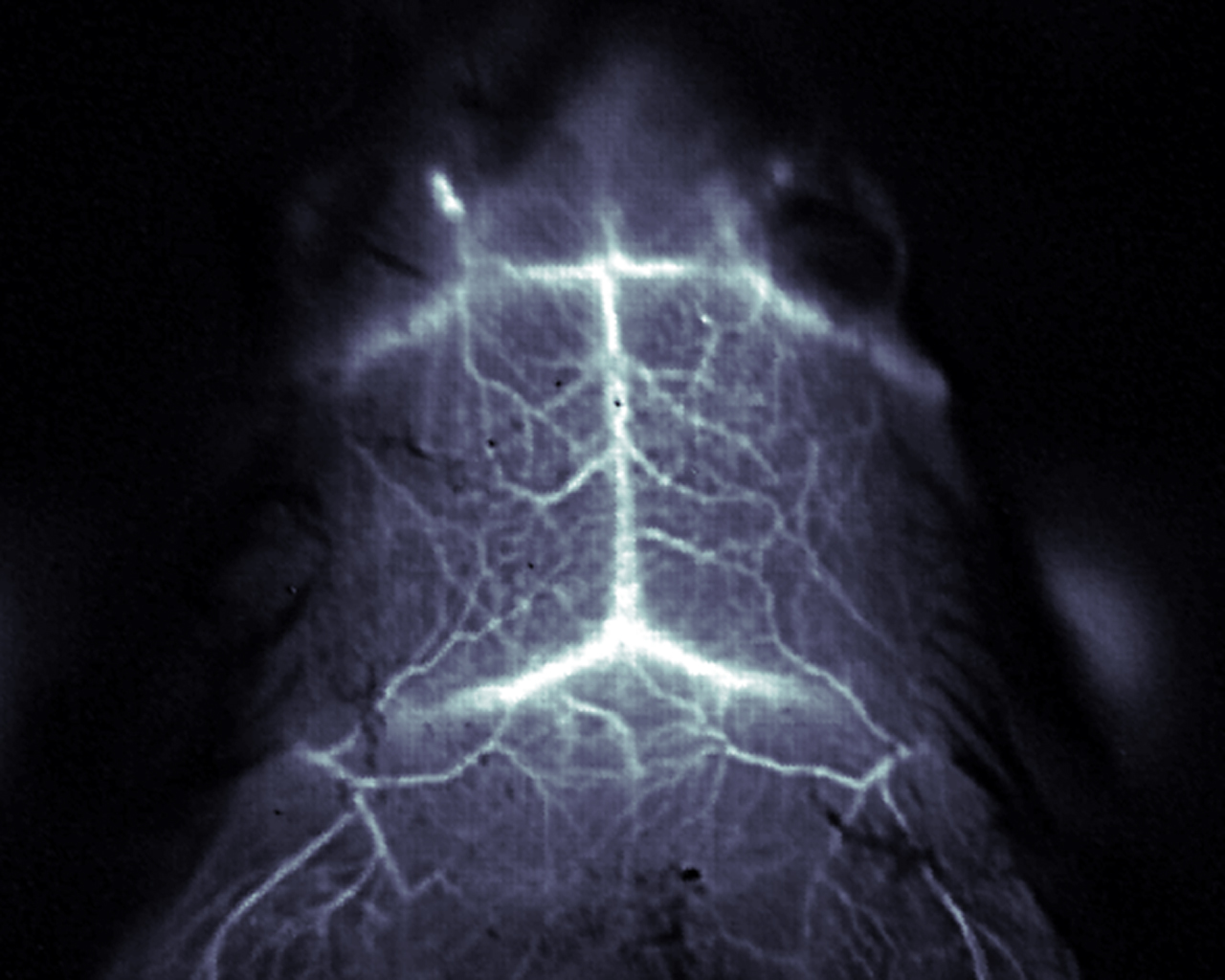
A fluorescent image of the mouse brain taken with a near-IR laser through the skull, clearly showing the brain vasculature of a live mouse through the intact scalp and skull (credit: Stanford Dai lab)
Stanford chemists have developed an non-invasive technique using lasers and carbon nanotubes to capture an unprecedented look at blood flowing through a living brain.
The new technique was developed for mice but could one day be applied to humans, potentially providing vital information in the study of stroke and migraines, and perhaps even Alzheimer’s and Parkinson’s diseases, the researchers say.
The work is described in the journal Nature Photonics.
The two main current procedures for exploring the brain in living animals both have issues:
- Surgically removing part of the skull offers a clear view of activity at the cellular level, but the trauma can alter the function or activity of the brain or even stimulate an immune response.
- Non-invasive techniques such as CT scans or MRI visualize function best at the whole-organ level, but they cannot visualize individual vessels or groups of neurons.
The new technique, called near infrared-IIa imaging (NIR-IIa*), overcomes these problems with a simple three-step procedure.
- Specially designed water-soluble carbon nanotubes are injected into a live mouse’s bloodstream.
- The researchers then shine near-infrared laser light (at 808 nm wavelength) into the brain from outside the skull.
- That light causes the nanotubes to fluoresce at wavelengths of 1,300–1,400 nanometers; this range represents a sweet spot for optimal penetration of the skull with very little light scattering, allowing for visualizing the blood vessels’ structure.
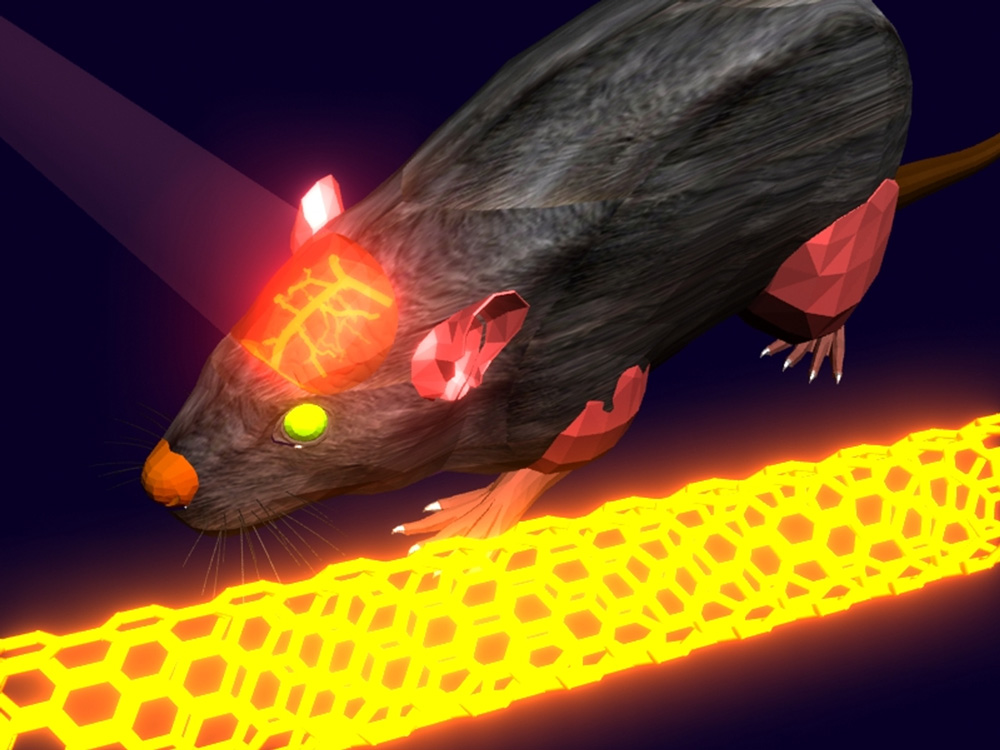
This illustration shows how single-walled carbon nanotubes, once injected into the subject, can be excited by near-infrared light at 808 nm and emit strongly in the 1300-1400 nm NIR-IIa window, allowing neuroscientists to visualize the brain vasculature and track cerebral blood flow through intact scalp skin and skull bone owing to the deep tissue penetration (credit: Dai lab)
The technique allows scientists to view about three millimeters underneath the scalp and allows for visualizing blood coursing through single capillaries only a few microns (millionths of a meter) across, said senior author Hongjie Dai, a professor of chemistry at Stanford.
The technique does not appear to have any adverse affect on innate brain functions.
The technique is similar to one called EROS used by researchers at Beckman Institute: they shine near-infrared light through the skull to detect neuron activity in the cortex. It’s done with humans and without a fluorescent agent, but does not provide a map of blood flow.
As reported by KurzweilAI in April, it’s based on the principle that when neurons are active, they swell a little, becoming slightly more transparent to light, indicating when a particular part of the cortex is processing information.
Human clinical trials
The Stanford technique could eventually be used in human clinical trials, said first author Guosong Hong, who conducted the research as a graduate student in Dai’s lab and is now a postdoctoral fellow at Harvard, but will need to be tweaked. The light penetration depth needs to be increased to pass deep into the human brain; and injecting carbon nanotubes needs approval for clinical application. The scientists are currently investigating alternative fluorescent agents.
For now, the technique provides a new technique for studying human cerebral-vascular diseases, such as stroke and migraines, in animal models. Other research has shown that Alzheimer’s and Parkinson’s diseases might cause (or be caused in part by) changes in blood flow to certain parts of the brain, Hong said, and NIR-IIa imaging might help better understand the role of healthy vasculature in those diseases.
“We could also label different neuron types in the brain with biomarkers and use this to monitor how each neuron performs,” Hong said. “Eventually, we might be able to use NIR-IIa to learn how each neuron functions inside of the brain.”
Researchers at Massachusetts General Hospital and Harvard Medical School were also involved in the study.
* NIR stands for “near-infrared” (to differentiate this light from longer-wavelength “mid-infrared” and “far-infrared” thermal radiation). Near-infrared is the light wavelength region longer than red light. The NIR-II region of near-infrared light is in the 1000 — 1700 nm wavelength region. It can reach deeper penetration depths in vivo than traditional NIR (750 — 900 nm, termed NIR-I). The NIRIIa region is from 1300 — 1400 nm.
Abstract of Nature Photonics paper
To date, brain imaging has largely relied on X-ray computed tomography and magnetic resonance angiography, with their limited spatial resolution and long scanning times. Fluorescence-based brain imaging in the visible and traditional near-infrared regions (400–900 nm) is an alternative, but at present it requires craniotomy, cranial windows and skull-thinning techniques, and the penetration depth is limited to 1–2 mm due to light scattering. Here, we report through-scalp and through-skull fluorescence imaging of mouse cerebral vasculature without craniotomy, utilizing the intrinsic photoluminescence of single-walled carbon nanotubes in the 1.3–1.4 μm near-infrared window (NIR-IIa window). Reduced photon scattering in this spectral region allows fluorescence imaging to a depth of >2 mm in mouse brain with sub-10-μm resolution. An imaging rate of ∼5.3 frames per second allows for dynamic recording of blood perfusion in the cerebral vessels with sufficient temporal resolution, providing real-time assessment of a blood flow anomaly in a mouse middle cerebral artery occlusion stroke model.