Using light to create a safer, more reliable pacemaker
September 26, 2011
Stanford University researchers have for the first time engineered human hearts to respond to light, in a breakthrough that could lead to biological rather than electrical pacemakers.
The pacemaker has served heart patients well over the years. Today, surgically implanted electrical pacemakers and defibrillators are commonplace, regulating the pulses of millions of faulty hearts around the globe. “But neither is without problems,” said Stanford research fellow Oscar Abilez, M.D.
“Pacemakers fail mechanically. The electrodes can cause tissue damage. Defibrillators, on the other hand,” said Stanford associate professor of mechanical engineering Ellen Kuhl, “can produce tissue damage due to the large electrical impulses that are sometimes needed to restore the heart’s normal rhythm.”
One method being explored is using light rather than electricity to set the pace for our tickers. Optogenetics involves genetically programming cells with proteins that are sensitive to light, so the heart cells can be activated by pulses of low-energy light. In the past few years, the technique has also been used on brain cells.
The latest breakthrough comes from Abilez, who has engineered human heart cells that can be paced with light using optogenetics.
Syncing the heart cells
The Stanford Researchers say this is the first time optogenetic beart pacing has been achieved, although a researcher at SUNY Stony Brook last month got some heart cells ticking using optogenetics as well. But the difference is this: the SUNY researcher, Emilia Entcheva, used donor cells engineered to be light-sensitive, which then acted as a kind of drum major — pulling the rest of the heart along to the rhythm.
What Abilez at Stanford did was engineer cardiomyocytes, actual heart cells that perform the drum-major function, to respond to light. In a key experiment, Abilez flipped a lever up and down, turning on and off a pale blue light aimed at a petri dish full of heart cells. Like a conductor, each time his finger went up, the heart cells contracted with the light.
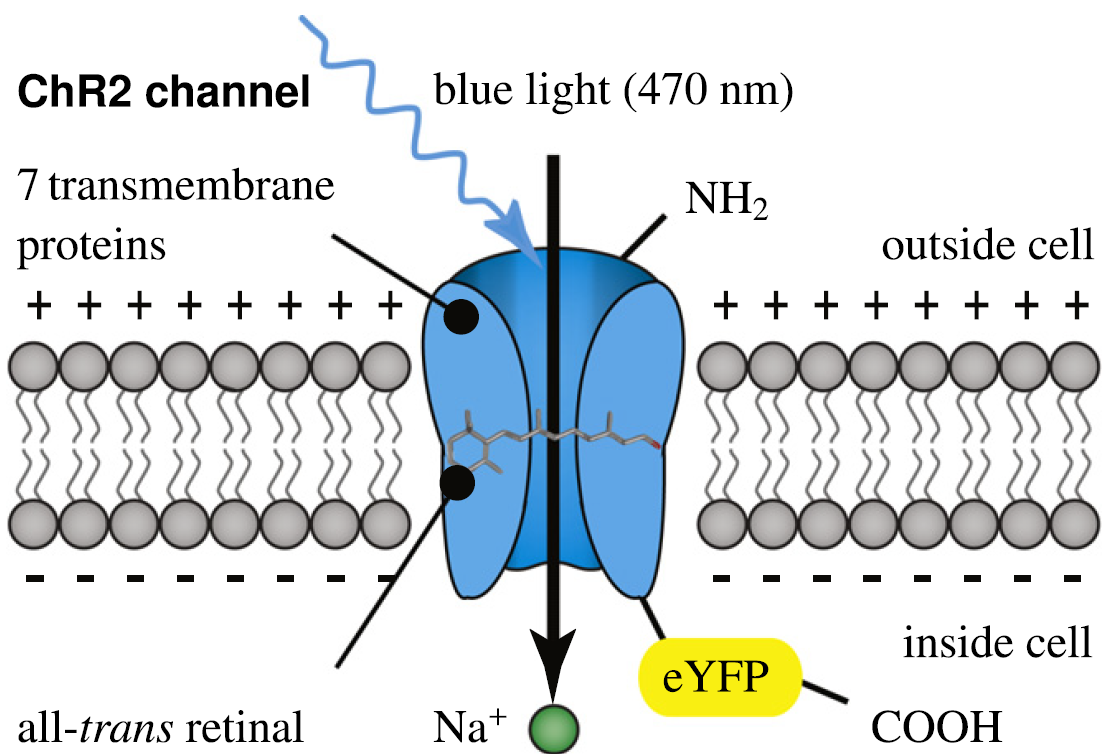
Channelrhodopsin-2 (ChR2) is a light-gated cation channel native to the green alga C. reinhardtii. It consists of seven transmembrane proteins and absorbs light through its interaction with retinal. Here, scientists induce channelrhodopsin coupled to enhanced yellow fluorescent protein (eYFP) into undifferentiated human embryonic stem cells via a lentiviral vector and differentiate these cells into cardiomyocytes. (Credit: Stanford University)
The heart cells were encoded to respond to light when the researchers inserted DNA, encoding a light-sensitive protein called channelrhodopsin-2 (ChR2) into human embryonic stem cells. ChR2 controls the flow of electrically charged ions into the cell.
For heart cells, the primary ion is sodium, which launches an electrochemical chain reaction that causes the cell to contract. Then they allowed the stem cells to grow into cardiomyocytes, which boasted the added feature of responding to light.
Future pacemakers
In the near term, the breakthrough will give researchers new insight into how the heart works or even be a decent research tool for those looking for possible cardiac side-effects to drugs being tested.
But in the long term, biological pacemakers could replace electrical ones. These biological pacemakers could be created from the patient’s own stem cells, so the body would not likely reject it. Or some day, the researchers say, there might be a pacemaker placed inside the heart chambers whose light can travel through the intervening blood to pace light-sensitive heart cells implanted inside.
“And, because the new heart cells are created from the host’s own stem cells, they would be a perfect genetic match,” Abilez said. “In principle, tissue rejection wouldn’t be an issue.”
The researchers caution, though, that a great many technical hurdles remain before light-based pacemakers will replace mechanical ones to create more reliable, less invasive devices.
Optogenetics could lead to advances beyond serving as a pacemaker, the researchers said. It might lead to new insights for treating cardiac arrhythmias, as well as various neuronal, musculoskeletal, pancreatic, and other disorders, including depression, schizophrenia, cerebral palsy, paralysis, diabetes, and pain syndromes.
Ref.: Oscar Abilez et al., Multiscale Computational Models for Optogenetic Control of Cardiac Function, Biophysical Journal, 2011; [in press]